|
Imaging Techniques Reveal that HIV Infects Host Cells Using a Molecular Entry Claw
|
|
|
NIH Press Release, May 3, 2007
An advanced imaging technique known as electron tomography has allowed researchers at the National Cancer Institute (NCI), part of the National Institutes of Health (NIH), to visualize an "entry claw," a unique structure formed between the human immunodeficiency virus (HIV) that causes AIDS and the cell it infects. The findings are in the May 4, 2007, issue of PLoS Pathogens.
"Visualizing the molecular mechanisms by which HIV and related viruses enter their host cells can potentially lead to the identification of novel drugs," said NIH Director Elias A. Zerhouni, M.D.
Retroviruses such as HIV establish contact and enter their target cells via an interaction between proteins on the surface of the virus (called spikes) and specific host cell membrane receptors. Previous studies have suggested that several spikes and several cell receptors are involved in every virus infection event. The findings of the NCI research team, led by Sriram Subramaniam, Ph.D., Laboratory of Cell Biology at NCI's Center for Cancer Research, demonstrate that this is true, but in a surprising way.
"This elegant research not only gives us insights into how HIV and related viruses interact with proteins on the surface of cells and then enter the host cells to integrate their DNA, it also gives us important clues as to how to design improved anti-HIV therapy," said NCI Director John E. Niederhuber, M.D. "Electron tomography and other new tools for imaging at the single-cell or subcellular level also have the potential to help us actually see the subcellular effects of many different diseases - including cancer - that we could only guess at previously."
In this study, the scientists showed that the interaction actually takes the form of a tight cluster of five to seven rod-shaped features. This striking and unexpected arrangement was dubbed the "entry claw" by the researchers (see figure below). They also found that the arrangement of spikes across the rest of the virus seems to largely disappear upon formation of the entry claw, suggesting a shedding event that has not previously been noted, which may have some, still uncertain relevance to infectivity.
"The discovery of the entry claw raises many fundamental questions about viral entry into host cells," said Subramaniam. "What are its precise molecular components? How does the viral genetic core actually transfer to the host cell? What are the other intermediate steps of the entry claw formation and can they be visualized? As we continue to improve the technology, we believe we will answer these and other related questions."
Traditional imaging techniques based on light microscopy do not offer sufficient resolution to see how cellular molecules and small structures interact in actual life, and powerful techniques such as X-ray crystallography can only define the structure of an individual molecule or simple molecular interactions. Electron tomography and related methods for 3D electron microscopy can fill this imaging gap and reveal fine subcellular structures or virus-host interactions in great detail. The Subramaniam lab has been pioneering advances in 3D electron microscopy, and is applying the emerging technologies to understanding not only virus-host interactions, but also visualizing such things as structures inside the cell that distinguish cancer cells from normal cells.
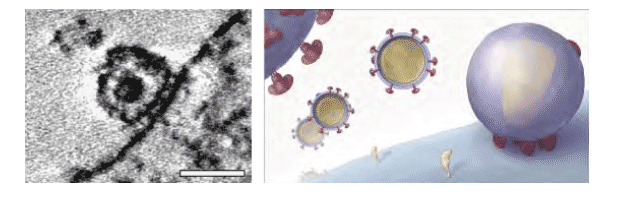
Contact of HIV-1 with T-cells. Electron tomographic analysis (left) reveals the architecture of the virus-cell contact region which forms an "entry claw" as shown in the schematic (right). Scale bar in left panel is 100 nanometers wide.
Reference: Sougrat R, Bartesaghi A, Lifson JD, Bennett AD, Bess J, Zabransky DJ, Subramaniam S. Electron tomography of the contact between T-cells and SIV/HIV-1: Implications for viral entry. PLoS Pathogens. May 4, 2007.
New HIV Entry Process Uncovered by Electron Tomography
Author Summary
Retroviruses such as simian immunodeficiency virus and HIV-1 enter target cells by exploiting the interaction between their surface glycoproteins and cell surface receptors. Knowledge of the structures of these glycoproteins and of the molecular details of their interaction with cell surface receptors is of fundamental interest in understanding viral entry mechanisms. Electron tomo-graphy is a powerful approach to determining the three-dimensional structures of large and heterogeneous sub-cellular assemblies such as virus-cell contact regions that cannot easily be analyzed by high-resolution structural methods such as X-ray crystallography. Here, we have used electron tomographic approaches to show that SIV and HIV-1 viruses make contact with T cells via a unique structure that we term the viral "entry claw", which is typically composed of about six clustered rods of density that span the contact region. Investigation of the structure of the entry claw and the factors that promote its formation could lead to new insights into the design of more effective drugs to inhibit HIV entry.
Introduction
Entry of HIV-1 into target cells involves the interaction of the surface glycoprotein gp120 (designated SU) with the cell surface receptor CD4 [1], a binding-induced structural change [2] in gp120 that creates the binding site for a cellular seven-transmembrane-helix co-receptor protein [3], followed by conformational changes [4] in the transmembrane glycoprotein gp41 (designated TM) that allow formation of the "pre-hairpin" conformation [5,6]. Insertion of the fusogenic portion of the TM polypeptide into the target cell membrane ultimately leads to fusion of the viral and target cell membranes [7]. Substantial insights into the entry mechanism have come from cell and structural biological studies, which suggest that the molecular species that initiates the steps leading to fusion is a trimer of envelope glycoprotein heterodimers [8].
Electron tomography is a powerful approach for determining the three-dimensional (3-D) structures of large and heterogeneous sub-cellular assemblies at resolutions that are typically one to two orders of magnitude higher than those that can be currently achieved using light microscopy [9-11]. Because these assemblies are not generally amenable to analysis by crystallographic approaches, electron tomography provides tools to bridge the gap between cellular and molecular structure. Although the highest resolutions have been obtained from tomographic analyses of thin, unstained specimens in a near-native state at cryogenic temperatures, significant information has also been derived from tomographic imaging of chemically fixed and stained specimens that allows structural investigation of the interior of thick cellular specimens. Here, we have used electron tomographic approaches to analyze the 3-D architectures of simian immunodeficiency virus (SIV) and HIV-1 virions incubated with CD4+ T lymphocyte target cells to identify structural features of cell-bound viruses trapped at a stage prior to entry into target cells.
Author Discussion
Viral binding and entry are key processes in the biology of AIDS virus infection and represent critical steps for potential prophylactic intervention by vaccine-induced neutralizing antibodies, or therapeutic intervention by inhibitors of binding or viral fusion. The observations we present here of the size and composition of the SIV and HIV-1 entry claw are in agreement with previous studies suggesting that viral entry is likely to be mediated by an oligomeric assembly of viral spikes and cell surface receptors. Experimental support for the cooperative interaction of multiple envelope glycoproteins in the entry of influenza [20], Semliki Forest virus [21], rabies virus [22], and baculovirus [23] has already been documented. In the case of HIV, there is also evidence that between four and six co-receptors (CCR5/CXCR4) and multiple CD4 molecules are likely to be present at the fusion pore [24,25]. The combination of these studies and the results of our structural analysis suggest that the entry claw is a specific macromolecular structure associated with initiating fusion and viral entry.
What stage of the fusion does the entry claw represent? Some models for viral fusion generally invoke the formation of a wide-necked pore via a hemi-fusion intermediate [26]. Despite thorough screening, we have not yet detected structures that appear to represent this type of fusion event where one might expect a partially fused virion in the act of transferring the viral genome-containing core into the cell. One possible explanation for this could be that the fusion event itself is very rapid, and therefore not detected in our experiments. In support of this idea, there is considerable evidence that once viruses bind to target cells, formation of sufficient numbers of ternary complexes of Env, CD4, and co-receptor is likely to be the rate-limiting step in viral entry [27]. This initial stage of viral-cell interaction corresponds at the biochemical level to generation of the pre-hairpin intermediate [6], and at the cellular level to the "temperature-arrested stage" [28], where viruses can remain attached to cells at temperatures that are suboptimal for fusion. In this model (Figure 7B), steps subsequent to initial docking must include, at a minimum (although not necessarily in this sequence), helix bundle formation, creation of the correct geometry for fusion, formation of a hemi-fusion intermediate leading to merger of the outer leaflets of the viral and target cell membranes, and the fusion event itself, which results in delivery of the viral genome into the cell.
There is an alternative model (Figure 7C) for how fusion might occur that is potentially consistent with recent studies that have concluded that a single fusion competent envelope glycoprotein trimer may be sufficient to support HIV-1 entry [29]. At first glance, these studies would appear to contradict the findings reported here, which consistently show multiple viral spikes acting in concert to form the entry claw. However, the key point is that in the analysis carried out by Sodroski and co-workers [29], the number of functional, fusion-competent trimers was modulated by varying the relative proportion of functional and defective (fusion-incompetent) glycoproteins present on surface of the virus, without alteration in the average number of spikes per virion. These non-functional envelope glycoproteins were mutants fully capable of CD4 binding, but defective for cleavage to generate fusogenic TM polypeptides. Thus, multiple trimers might participate in formation of an entry claw structure, creating a stabilized scaffold to dock the virus to the cell membrane, regardless of their fusion competence. If fusion were to occur via local puncture of the membrane at the location of the single functional spike, presumably via the formation of a fusogenic, six-helix bundle intermediate [6], it could account both for the structural role of multiple viral spikes in forming the claw to create the scaffold to dock the virus to the cell membrane, and the finding that one functional trimer is adequate for viral entry. The electron tomographic analyses show that dimensions of the pore that could be formed between the anchors of the claw could be as wide as ~300 A. An opening of this size is comparable to the dimensions of an intact viral core [30] and may thus be large enough to transport the core of the virus into the cell even without invoking any additional pore expansion. In this mechanism, the entry claw scaffold and the viral envelope could continue to remain attached on the cell surface after loss of viral core. Although evidence of staining from viral cores was observed in entry claw contacts after both short and long incubation periods, at present, we cannot definitively distinguish between the two models in Figure 8.
The discovery of the entry claw raises many fundamental questions about viral entry. What is the molecular composition and stoichiometry of the components forming the entry claw? Do other complex factors such as membrane reorganization and actin rearrangement play a role in its formation or stabilization? Is persistence of the entry claw dictated by the speed of the topological rearrangements needed for formation of the helix bundle that triggers membrane fusion? Does the composition of the claw change following initial contact? Are there other structural intermediates that can be trapped by using selected gp120 or gp41 mutants with altered functional properties, or by the addition of antibodies or ligands to the cell surface receptors involved? Can the fusion event itself be captured and visualized? What is the role, if any, of interactions between the C-terminal tail of the envelope glycoprotein (TM) and the Gag matrix in rearrangements of viral spikes at the point of contact? Continuing improvements in technologies for cryo-electron tomography, combined with X-ray crystallographic analysis of the envelope glycoprotein in its various conformations, offer the promise of making significant advances towards answering these questions.
|
|
|
|
|
|
|