|
Study unpicks how AIDS causes dementia
|
|
|
HIV triggers the 'opposite of cancer' in the brain
Nature.com
Published online: 15 August 2007
Ewen Callaway
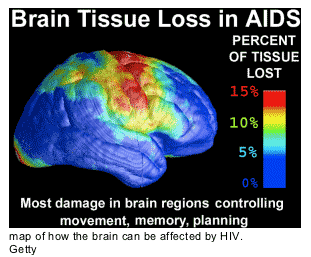
A study showing how HIV could prevent the brain from making new neurons offers an explanation for why some AIDS patients get dementia - and suggests a possible treatment.
Dementia due to HIV is the leading cause of cognitive decline in people under 40 years of age, says Stuart Lipton, a biologist at the Burnham Institute for Medical Research in La Jolla, California, who led the study in Cell Stem Cell1. Researchers aren't sure what causes the condition, which afflicts 10-30% of people with HIV and causes symptoms including forgetfulness and leg weakness. If untreated with antiretroviral drugs, sufferers can turn comatose.
Biologists have two theories to explain AIDS-related dementia. It could be that when HIV infects a type of white blood cell called a macrophage, the cell pumps out inflammatory chemicals to battle the infection that also, unfortunately, wipe out neurons.
Or HIV could inflict its damage more directly. One previous study showed that a protein in the virus's shell - called gp120 - can stop brain stem cells from dividing2. Such new stem cells are needed to make new neurons.
Undivided
To investigate, Lipton and postdoc Shu-ichi Okamoto studied a strain of mice genetically engineered to make the virus's gp120 protein. Under the microscope, the mouse brains look just like those of humans with AIDS-related dementia, says Lipton.
"This is the first hint at how HIV can co-opt stem cells and cause them to dysfunction."
Stuart Lipton
Burnham Institute for Medical Research
When the team studied the mice more closely, they found many of their neural stem cells were stuck in the cellular equivalent of 'neutral', unable to divide and make new cells.
Neural stem cells in the transgenic mice also contained more of a protein called p38 than normal mice. In healthy cells, p38 guards against cancer by halting cell division when DNA strands get broken. If HIV prompts so much p38 that it stops normal cells from dividing, "it's the opposite of cancer", says Lipton. The researchers also found other proteins linked to p38 in the neural stem cells of the gp120-expressing mice.
"This is the first hint at how HIV can co-opt stem cells and cause them to dysfunction," says Lipton.
Chemical cure
Drugs that block p38 are already in clinical trials for autoimmune diseases such as rheumatoid arthritis, and Lipton's team is currently testing one such drug in mice as a possible treatment for AIDS-related dementia.
At present, antiretroviral drugs are sometimes helpful at preventing dementia, but some do a better job than others, and patients eventually become resistant to the drugs. A different way of treating the condition would be useful patients who don't respond to antiretrovirals, says Francisco Gonzales-Scarano, a neurologist at the University of Pennsylvania in Philadelphia. "At this point there really aren't any [such treatments]," he says.
But Gonzales-Scarano wonders whether there is really enough viral protein in the brain of AIDS patients to cause dementia. If not, some other, less direct mechanism could be to blame for the problem.
References
1. Okamoto, S. et al. Cell Stem Cell 1, 230-236 (2007).
2. Krathwohl, M. D. & Kaiser, J. L. Infect. Dis. 190, 216-226 (2007).
"HIV/gp120 Decreases Adult Neural Progenitor Cell Proliferation via Checkpoint Kinase-Mediated Cell-Cycle Withdrawal and G1 Arrest"
Cell Stem Cell, Vol 1, 230-236, 16 August 2007
Shu-ichi Okamoto,1,* Yeon-Joo Kang,1,4 Christopher W. Brechtel,1,2,4 Elisa Siviglia,1,4 Rossella Russo,1 Arjay Clemente,1 Anne Harrop,1 Scott McKercher,1 Marcus Kaul,1,3,** and Stuart A. Lipton1,2,3,***
1 Center for Neuroscience, Stem Cells, and Aging, Burnham Institute for Medical Research, La Jolla, CA 92037, USA
2 Department of Neurosciences, University of California, San Diego, La Jolla, CA 92093, USA
3 Department of Psychiatry University of California, San Diego, La Jolla, CA 92093, USA
SUMMARY
Impaired adult neurogenesis has been observed in several neurodegenerative diseases, including human immunodeficiency virus (HIV-1)-associated dementia (HAD). Here we report that the HIV-envelope glycoprotein gp120, which is associated with HAD pathogenesis, inhibits proliferation of adult neural progenitor cells (aNPCs) in vitro and in vivo in the dentate gyrus of the hippocampus of HIV/gp120-transgenic mice. We demonstrate that HIV/gp120 arrests cell-cycle progression of aNPCs at the G1 phase via a cascade consisting of p38 mitogen-activated protein kinase (MAPK) → MAPK-activated protein kinase 2 (a cell-cycle checkpoint kinase) → Cdc25B/C. Our findings define a molecular mechanism that compromises adult neurogenesis in this neurodegenerative disorder.
INTRODUCTION
HIV infection causes neurodegeneration accompanied by progressive motor and cognitive dysfunctions, designated HIV-associated dementia (HAD) (Gonzalez-Scarano et al., 2005, Kaul et al., 2005). The prevalence of HAD is rising despite highly active antiretroviral therapy (HAART) because patients survive longer and these drugs have limited penetrance into the brain; persistent exposure of the central nervous system to HIV is a major risk factor for HAD (Gonzalez-Scarano et al., 2005, Kaul et al., 2005). Recent evidence has shown that HAD patients manifest fewer adult neural progenitor cells (aNPCs) in the dentate gyrus of the hippocampus, an important center for memory and learning, than noninfected subjects or HIV-infected patients without dementia (Krathwohl et al., 2004, Krathwohl et al., 2004). The dentate gyrus contains excitatory granule cell neurons, which receive inputs from the entorhinal cortex and project to the CA3 region of the hippocampus. Normally, neurogenesis occurs constitutively in the dentate gyrus throughout adulthood (Aimone et al., 2006, Lledo et al., 2006). Newly generated neurons are electrophysiologically plastic and functionally integrate into existing circuits (Aimone et al., 2006, Lledo et al., 2006). This maturation process is modulated by neuronal activity via neurotransmitters such as GABA and glutamate (Ge et al., 2006, Tashiro et al., 2006, Tozuka et al., 2005). Young neurons are more excitable than their neighboring, more mature granule cell neurons (Kee et al., 2007, Ramirez-Amaya et al., 2006). Moreover, the newborn neurons are thought to contribute to certain forms of learning and memory (Aimone et al., 2006, Lledo et al., 2006). Intriguingly, hippocampal neurogenesis has been reported to be dysregulated in several neurodegenerative conditions, including Alzheimer's and Huntington's diseases (Ming et al., 2005). Nonetheless, the molecular mechanism underlying this dysfunction in adult neurogenesis remains unknown. Here we report that activation of the p38 mitogen-activated protein kinase (MAPK) → MAPK-activated protein kinase 2 → Cdc25B/C cascade decreases proliferation of aNPCs in a murine model of HAD and neurodegeneration.
RESULTS
HIV-1 Envelope Glycoprotein gp120 Decreases Proliferation of Adult Neural Progenitors In Vitro and In Vivo
The development of HAD is ascribable to multiple host cell-derived products and viral proteins, such as the HIV-1 envelope glycoprotein gp120 (Gonzalez-Scarano et al., 2005, Kaul et al., 2005). HIV-1 infects predominantly microglia in the brain, and the gp120 envelope protein can be shed from HIV and diffuse through the extracellular space (reviewed in Kaul et al., 2005). We and others have demonstrated that gp120 plays a pivotal role in the pathogenesis of HAD through CXC chemokine receptor 4 (CXCR4) and CC chemokine receptor 5 (CCR5) (Hayward, 2004, Kaul et al., 2005, Tran et al., 2003), both of which are expressed in aNPCs (Tran et al., 2004). We recently showed that the preference of gp120 for human CXCR4 or CCR5 also holds true for these chemokine receptors in rodents (Kaul et al., 2007). To investigate the potential contribution of HIV-1 to perturbed neurogenesis in HAD patients (Krathwohl et al., 2004, Krathwohl et al., 2004), we initially studied the effect of gp120 protein on adult rat hippocampal neural progenitors, whose homogeneity and multipotency have been well characterized (Gage et al., 1995, Kuwabara et al., 2004, Lie et al., 2005, Muotri et al., 2005, Palmer et al., 1997, Song et al., 2002). First, we evaluated the effect of gp120 on the survival of these aNPCs because gp120 has been shown to induce neuronal cell death (Garden et al., 2002, Kaul et al., 1999). Cell death versus survival was evaluated based on propiduim iodide uptake, cell morphology, DNA condensation, and TUNEL staining in aNPC cultures exposed to increasing amounts of gp120 protein. Unexpectedly, at concentrations known to induce apoptosis in neurons in our laboratory and others (Kaul et al., 1999), gp120 did not promote apoptotic death of aNPCs when measured at 1 day (Figure 1A) or 3 days (Figure S1A in the Supplemental Data available with this article online). As a positive control, exposure to staurosporine did induce apoptosis within 24 hr (Figure 1A). Next, necrosis was assessed by using the DNA-binding dye propidium iodide. Propidium iodide is excluded from both live and apoptotic cells and is incorporated into necrotic cells when membrane integrity is lost. Figure S1B demonstrates that necrotic cell death was not observed at 1 day or 3 days post-gp120 insult, whereas 3 mM hydrogen peroxide significantly induced necrosis at 1 day. Taken together, these findings indicate that gp120 does not affect survival of aNPCs by inducing apoptosis or necrosis.
Next, we asked if gp120 had an effect on aNPC proliferation as assessed by Ki-67 staining (Chenn et al., 2002); gp120 significantly decreased the number of Ki-67-positive progenitors, whereas vehicle control or heat-inactivated gp120 did not (Figure 1B). In conjunction, these data indicate that gp120 decreases the fraction of aNPCs that are actively proliferating without inducing cell death. The pathophysiological relevance of these in vitro findings was further evaluated in vivo in transgenic mice specifically expressing gp120 in the brain; these mice recapitulate several features of the neuropathology seen in human HAD patients (Garden et al., 2002, Toggas et al., 1994). To analyze proliferation of progenitors in the dentate gyrus, 5-bromo-2-deoxyuridine (BrdU) was injected intraperitoneally for 10 consecutive days, and subsequently, BrdU-positive cells in the dentate gyrus were counted. We observed a significant decrease in the number of proliferating cells in gp120-transgenic compared to wild-type littermate mice (Figure 1C and 1D). BrdU-labeled cells (red) are negative for the glial marker S100β (blue) (Figure 1C) but positive for the progenitor marker PSA-NCAM (Figure 1E). These results suggest that the BrdU-positive cells are neural progenitors rather than proliferating glia. Next, we addressed the possibility of apoptosis occurring in vivo in response to gp120. TUNEL analysis revealed virtually no apoptotic cells in the dentate gyrus in control- or gp120-transgenic mice (Figure S2). Together, these findings show that gp120 decreases the number of aNPCs both in vitro and in vivo by inhibiting proliferation rather than increasing cell death.
Effect of gp120 on Cell-Cycle Kinetics of Adult Neural Progenitors
Next, we investigated the mechanism of gp120 reduction in aNPC proliferation. Cell-cycle kinetics were analyzed in cultured aNPCs. Cumulative S phase labeling with BrdU was used to measure cell-cycle parameters, including the proportion of proliferating cells, the duration of the cell cycle, and the lengths of G1, S, G2, and M phases (Figure 2A) (Takahashi et al., 1993). We observed that gp120 lengthened the total cell cycle, especially in G1 (Figure 2B). Moreover, the maximum proportion of BrdU-positive aNPCs was significantly decreased after gp120 exposure (87.7%) compared to control (95.3%; p ≦ 0.003; Figure 2A), suggesting that progression into S phase was delayed.
We then determined if gp120 arrests the proliferation of aNPCs in G1 phase at the end of each round of division. aNPCs in S phase were pulse labeled with BrdU for 3 hr. After washing out the BrdU, cells were cultured for 9 additional hr. Based on the cell kinetics (Figure 2B), 9 hr was sufficient for all BrdU-labeled cells to exit M phase, remain in G1 phase, but not yet re-enter S phase. Cells were subsequently exposed to vehicle or gp120 for an additional 14-18 hr, and progression through another cell cycle was determined by staining with the proliferation marker Ki-67 (Chenn et al., 2002). Ki-67/BrdU double-labeled cells were then compared to the total number of BrdU-positive cells. The double-positive cells were scored as the fraction that progressed through a second cell cycle. As shown in Figure 2C, 14 hr after gp120 exposure, 71% of the cells were Ki-67/BrdU double positive, whereas 96% were doubly labeled in control cultures. After a 16 hr incubation with gp120, the proportion of Ki-67/BrdU double-positive cells reached 85%, which did not further increase after 18 hr. These results suggest that gp120 prolonged G1, thereby arresting the cell cycle.
Cell-cycle withdrawal occurs during the process of cellular differentiation. Because we observed that gp120 modulated both the length and rate of the cell cycle of aNPCs, we asked whether their ability to differentiate would also be affected. For this experiment, we probed progenitor cells with antibodies to βIII-tubulin (TuJ1) and S100β-early neuronal and astroglial markers, respectively. We observed no difference in the number of cells expressing either marker after exposure to gp120 or control (Figure S3). This finding suggests that the gp120-induced reduction in proliferation is not likely to result in premature neuronal or astroglial differentiation.
gp120 Evokes the p38 MAPK-MAPKAPK2-Cdc25C Cascade
Previously, effects of gp120 on cell-cycle-associated molecules had been described in postmitotic neurons (Jordan-Sciutto et al., 2002, Khan et al., 2003). Additionally, activation of p38 MAPK was known to affect the cell cycle (Takenaka et al., 1998, Manke et al., 2005). Furthermore, we and others had demonstrated that gp120 activates p38 MAPK in mature neurons (Kaul et al., 1999, Singh et al., 2005). Hence, we asked if a similar signaling pathway might be responsible for the decrement in cell proliferation of aNPCs induced by gp120. To test this idea, cell extracts were prepared from aNPC cultures exposed to gp120 or vehicle. Western blotting with an antibody against phosphorylated (activated) p38 MAPK revealed a dramatic increase in enzyme activation after gp120 exposure (Figure 3A). The phospho-p38 antibody used in the present study recognizes all phosphorylated p38 isoforms (Cell Signaling). However, the various p38 isoforms differ in molecular weight so the identity of the activated isoform can be distinguished by western blot. We found that the phosphorylated p38 band was identical to p38 in mobility, indicating that p38 is the isoform activated by gp120.
An inhibitory effect of p38 MAPK on cell-cycle progression had been previously demonstrated in other cell types (Takenaka et al., 1998). In fact, p38 MAPK-by phosphorylating MAPKAP kinase 2 (MAPKAPK2), which in turn phosphorylates and inactivates Cdc25C-was recently shown to underlie a DNA damage-induced checkpoint pathway involving G1 phase arrest (Manke et al., 2005). To determine whether this cascade is activated in aNPCs exposed to gp120, we examined the phosphorylation patterns of MAPKAPK2 and Cdc25C in extracts from progenitor cultures. We observed increased phosphorylation of MAPKAPK2 and Cdc25C under these conditions (Figure 3A). Another Cdc25 family member, Cdc25A, has been reported to be the Cdc25 family member predominantly controlling G1/S transition. The mode of inactivation of Cdc25A has been described to be proteasome-dependent degradation after phosphorylation (Mailand et al., 2000). Therefore, we also examined Cdc25A levels (Figure 3A and Figure S4); however, gp120 treatment did not decrease Cdc25A protein, suggesting that Cdc25A is not involved in the gp120 effect observed here.
Notably, we observed that the classical pathway to DNA damage-induced checkpoint activation, involving ATM-Chk1/2, was not activated by gp120, although as a positive control the genotoxin etoposide induced activation/phosphorylation of ATM-Chk1/2 (Figure 3B). Altogether, these results suggest that gp120 activates a p38 MAPK-MAPKAPK2-Cdc25C cascade in aNPCs.
The p38 MAPK-MAPKAPK2-Cdc25B/C Pathway Impairs Proliferation of Adult Neural Progenitors
Next, we asked whether activation of the p38 MAPK-MAPKAPK2-Cdc25C pathway mediates the gp120-induced decrease in proliferation of aNPCs. Similar to gp120, we found that overexpression of wild-type p38 MAPK resulted in a decrease in the fraction of Ki-67-positive cells (Figure 4A), indicating a decrease in proliferation. Conversely, transfection of a dominant-negative form of p38 MAPK (p38DN) (Han et al., 1994) abrogated the gp120-induced decrease in aNPC proliferation (Figure 4A). Additionally, the role of MAPKAPK2 was analyzed by using RNA interference (RNAi) techniques. Transfection of aNPCs with MAPKAPK2 sh (small hairpin) RNA vector-1 or -2 resulted in a substantial reduction in MAPKAPK2 protein levels within 48 hr (Figure S5). Expression of each MAPKAPK2 shRNA rescued the gp120-dependent decrease in proliferation (Figure 4B), consistent with the notion that the p38 MAPK-MAPKAPK2 axis mediates the inhibitory effect of gp120 on progenitor proliferation. MAPKAPK2 is known to reduce cell-cycle progression via inactivation of Cdc25C by phosphorylation of Ser-216 (Manke et al., 2005). We found that the gp120-induced increase in Ser-216 phosphorylation of Cdc25C was reduced by the p38DN (Figure S6A) or MAPKAPK2 shRNAs (Figure S6B), suggesting that Cdc25C is the downstream effector of p38 MAPK-MAPKAPK2. To test this premise further, we constructed a Cdc25C-S216A mutant (Cdc25Cmt) previously shown to be resistant to phosphorylation-dependent inactivation (Smits et al., 2000), hypothesizing that this mutant would abrogate the inhibition of cell-cycle progression induced by gp120. Indeed, we found that transfection of aNPCs with Cdc25Cmt reduced gp120-initiated inhibition of cell proliferation (Figure 4C).
In addition to Cdc25C, Cdc25B is phosphorylated and inactivated by MAPKAPK2 (Manke et al., 2005). To determine if inhibition of Cdc25B and/or Cdc25C is responsible for arrest in G1, we generated two shRNA vectors specific for Cdc25B and two additional shRNA vectors specific for Cdc25C in order to examine the selective effects of each of these Cdc25 isoforms on BrdU uptake. Accordingly, we show that Cdc25B shRNAs efficiently knocked down levels of Cdc25B but not Cdc25C protein. Conversely, Cdc25C shRNAs knocked down Cdc25C but not Cdc25B protein (Figure S7A). All four shRNA constructs significantly reduced BrdU uptake (Figure S7B). These findings suggest that both Cdc25 isoforms may contribute to the effect, and thus a p38 MAPK-MAPKAPK2-Cdc25B/C cascade mediates the inhibitory effect of gp120 on aNPC proliferation.
DISCUSSION
HIV-1 envelope protein gp120 has been shown to play an important role in the pathogenesis of HAD via interaction with chemokine receptors CXCR4 and CCR5 (Hayward, 2004, Kaul et al., 2005, Tran et al., 2003). Here, we show that gp120 arrests proliferation of aNPCs in G1 phase and define the molecular pathway underlying this effect in rodent models. Although a direct interaction between gp120 and CXCR4 or CCR5 has not yet been demonstrated in the rodent central nervous system, we recently reported that concentrations of gp120 as low at 200 pM exert neurotoxic effects via these chemokine receptors (Kaul et al., 2007). Importantly, we also found that the preference of a given gp120 for CXCR4 or CCR5 was the same in human and mouse cells. Thus, a particular gp120 that has been classified as CCR5 preferring for human cells also interacts predominantly with mouse CCR5, and similarly, a gp120 preferring human CXCR4 also prefers murine CXCR4 (Kaul et al., 2007). Thus, the molecular dissection of chemokine receptor-triggered pathways in the present study using rodent models should have pathogenic relevance for the dysregulation of adult neurogenesis in HAD patients.
Further supporting the pathophysiological relevance of our model systems, we found a significant decrement in the number of aNPCs in the dentate gyrus of HIV/gp120-transgenic mice similar to that previously observed in the brains of HAD patients (Krathwohl et al., 2004, Krathwohl et al., 2004). Adult neurogenesis has been postulated to be important for the processes of learning and memory (Aimone et al., 2006, Lledo et al., 2006) and is thus relevant to HAD. Moreover, the CXCR4 chemokine receptor has been shown to be critical for the development of the hippocampal dentate gyrus (Lu et al., 2002), and gp120 interferes with CXCR4-mediated signaling in aNPCs (Tran et al., 2005). Thus, development and maintenance of the dentate gyrus could potentially be perturbed in gp120-transgenic mice. Although the width of the NeuN (neuronal marker)-positive band in the dentate gyrus did not appear to be reduced in gp120-transgenic mice (Figure 1C), detailed stereological counting of neurons will be needed to demonstrate if fewer neurons are present in these mice.
Most importantly, the present study provides mechanistic insight into the inhibition of aNPC proliferation by HIV/gp120 via activation of the p38 MAPK-MAPKAPK2-Cdc25B/C cascade. Previously, activation of the p38 MAPK pathway in postmitotic, mature neurons had been shown to promote cell cycle re-entry and subsequent apoptosis (Zhu et al., 2004). Intriguingly, therefore, p38 MAPK appears to contribute to dysregulation of adult neurogenesis and degeneration of postmitotic neurons in a diametrically opposed manner; cell-cycle machinery is inhibited in neural progenitors while activated in mature neurons. The p38 MAPK-MAPKAPK2-Cdc25B/C cascade has also been reported to mediate a DNA damage-induced checkpoint pathway (Manke et al., 2005). Checkpoint activation has been intensively studied in cancer biology as a means to inhibit cell-cycle progression, allowing genomic repair to be accomplished. Now we show that a similar pathway may account for gp120-induced G1 arrest of aNPCs. This finding, however, indicates that the p38-MAPKAPK2-Cdc25B/C cascade, in addition to being a DNA damage-dependent checkpoint pathway, appears to affect cell-cycle kinetics in a DNA damage-independent fashion by controlling exit from G1. Because decreased proliferation of aNPCs has been observed in patients with HAD, this same mechanism may be detrimental to the development of new neurons in HIV-associated and other neurodegenerative conditions. In support of this premise, others have reported dysregulation of the p38 MAPK signaling pathway and aberrant neurogenesis in various neurodegenerative diseases (Harper et al., 2003, Klein et al., 2003, Ming et al., 2005). Our findings thus provide a new perspective for understanding the molecular mechanism underlying aberrant adult neurogenesis observed in the brain during neurodegenerative disorders.
In summary, in response to gp120, rat aNPCs manifest a defect in proliferation in vitro. In addition, this proliferation defect in aNPCs is observed in the HIV/gp120 transgenic mouse model in vivo. Besides reduced proliferation, gp120 induces slowed cell-cycle kinetics, primarily due to a prolonged G1 phase. In response to HIV/gp120, the p38 MAPK/MAPKAPK2 signaling complex is activated. Furthermore, in response to gp120, the critical cell-cycle checkpoint target Cdc25C becomes phosphorylated while the canonical checkpoint cascades ATM/Chk2 and ATR/Chk1 appear to be inactive. In the absence of genomic damage, this decrease in proliferation, rather than reflecting a true"checkpoint," at least in its traditional sense, may represent a cellular stress response. Thus, our new findings suggest that the p38 MAPK/MAPKAPK2 cascade, in addition to being a DNA damage-dependent checkpoint pathway, also appears to affect cell-cycle kinetics in a DNA damage-independent fashion by controlling exit from G1.
|
|
|
|
|
|
|