|
Clinical Use of Serum and Urine Bone Markers in the Management of Osteoporosis
|
|
|
Curr Med Res Opin. 2005;21(7):1015-1026.
Apurva K. Srivastava; Elizabeth L. Vliet; E. Michael Lewiecki; Michael Maricic; Alex Abdelmalek; Oscar Gluck; David J. Baylink
a Musculoskeletal Disease Center, VA Loma Linda Healthcare System, and Department of Medicine, Loma Linda University, Loma Linda, CA, USA
b New Mexico Clinical Research & Osteoporosis Center, Inc., Albuquerque, NM, USA
c Preventive and Climatic Medicine, Health Enhancement Renewal for Women, Inc., Tucson, AZ, USA
d Section of Rheumatology of Primary Care at the Southern Arizona VA Health Care System, and Clinical Medicine at the University of Arizona School of Medicine, Tucson, AZ, USA
e Kaiser Permanente, West Los Angeles and Department of Medicine at University of California at Los Angeles, Los Angeles, CA, USA
University of Arizona, Director Arizona Rheumatology Center, Phoenix, AZ, USA
Declaration of interests: There are no financial or proprietary relationships to report regarding this review. Loma Linda University received a small unrestricted grant from the Alliance for Better Bone Health (A Partnership between Procter & Gamble Pharmaceuticals and Sanofi-Aventis Pharmaceuticals) to cover administrative costs for manuscript preparation. Authors were not compensated and no comments or editorial support were provided by industry.
ABSTRACT
Osteoporosis is a common disease characterized by decreased bone mass, increased bone turnover, and increased susceptibility to fracture. Almost 44 million Americans are estimated to have low bone mass, which puts them at increased risk of developing osteoporosis and fractures. Osteoporosis is diagnosed by a low bone density (BMD) measurement, because a low BMD is known to contribute to increased fracture risk, which is the main source of morbidity and mortality for osteoporosis. However, changes in bone mass and density in response to anti-resorptive therapy account for only a small portion of the predicted fracture risk reduction. Whereas dynamic changes in bone turnover, estimated by measurement of bone biochemical markers, such as breakdown products of type-I collagen and proteins secreted by osteoblasts and osteoclasts in blood and urine, can account for a major portion of anti-fracture efficacy of anti-resorptive agents. Most anti-resorptive agents act by rapidly reducing bone markers. This has led to advocacy for use of bone turnover markers, in complement to BMD measurement, in the management of osteoporosis. In general, higher bone turnover is associated with accelerated bone loss and potential deterioration in bone quality. Several clinical trials have established the potential utility of markers to identify patients with rapid bone loss, to aid in therapeutic decision-making, and to monitor therapeutic efficacy of various treatments. Elevated marker levels have been shown to be associated with increased risk of fracture in elderly women, but their utility in predicting fracture is not yet established. In this article, we provide a brief summary to primary practitioners about the role bone markers can play in the management of osteoporosis.
INTRODUCTION
Osteoporosis is a major public health threat for an estimated 44 million Americans with direct national expenditure of $17 billion in 2001.[1] In the US today, 10 million individuals are estimated to already have the disease and this figure is expected to climb to over 17 million by 2010 and 20 million by 2020. Of the 10 million Americans estimated to have osteoporosis, 8 million are women and 2 million are men. In addition, nearly 34 million Americans are estimated to have low bone mass, which puts them at increased risk of developing osteoporosis and related fractures.
Osteoporosis is defined as a skeletal disorder characterized by compromised bone strength[2] predisposing to an increased risk of fracture. Consequently, deterioration in bone strength and fragility, in addition to decrease in bone mass and density, have been identified as factors that increase fracture risk. The diagnosis of osteoporosis is made by determining that the patient has a low bone density, usually by a Dual Energy X-ray Absortiometry[3] (DEXA) measurement. This is important because a low bone density places a patient at increased risk for fracture,[4,5] which is the ultimate adverse effect and main source of morbidity and mortality from osteoporosis disease. However, BMD is only one of the contributors to bone strength. The other main determinant of bone strength is derived from bone quality, which consists of bone mass, size, structure (micro-and macro-architecture), material properties, and bone remodeling.[6] The issue of bone quality has recently received increasing attention because data from large clinical trials of anti-resorptive drugs have indicated that reduction in fracture risk or improvement in bone quality evoked by these drugs are only partly explained by increase in BMD.[7,8] Several recent studies[6,9-14] indicate a paradigm shift in exploring the underlying basis of this inconsistency by providing evidence that elevated bone remodeling activity accounts for a major fraction of both reduction in fracture risk and improvement in bone quality.[6,9-12,15] In this regard, measurement of proteins and peptides, referred to as biochemical bone markers, that are released as a consequence of the physiological action of osteoblasts and osteoclasts provide the most practical tool currently available to measure bone remodeling in routine clinical practice. These bone biochemical markers can be found in the serum and urine and are categorized as markers of bone formation or bone resorption.[16] It is of particular interest that measurement of bone biochemical markers may provide some assessment of factors that contribute to bone quality because the bone biochemical markers measure remodeling and, in general, a higher remodeling rate is detrimental to bone quality.[15] Therefore, the best assessment of fracture risk may be made by bone density measurement along with the measurement of bone biochemical markers.
The remodeling or bone turnover occurs via a coupled process of bone resorption and bone formation. Bone resorption and bone formation are accomplished in a finely orchestrated sequence, initiated by osteoclasts, in front, followed by osteoblasts in a unit called 'basic multicellular unit' (BMU) [as shown in Figure 1(A-D)]. Individual BMUs maintain their integrity for approximately 4-8 months, with a range of 3 months-2 years[17] It is believed that approximately one million BMUs are thought to be functioning in a healthy adult skeleton at any given time, replacing about 8%-10% of bone tissue annually, presumably to avoid loss of material properties and microdamages due to aging.[18] In cancellous bone, BMUs create trenches as they move across the surface; in cortical bone, BMUs move through the bone matrix increasing porosity. During bone resorption, osteoclasts create resorption pits with low pH microenvironments, which dissolve the inorganic matrix exposing the organic matrix (> 90% of the organic matrix is composed of type-I collagen). Subsequently, bone-reabsorbing enzymes digest the organic bone matrix releasing breakdown products of type-I collagen, which include terminal peptide fragments from both ends of the type- I collagen such as N-terminal telopeptides called NTx,[19] C-terminal telopeptides called CTx,[20] and ring structures collectively called pyridinium crosslinks.[21] Tests are now available that can specifically measure these collagen breakdown products. In healthy bone, the resorption cavity created by osteoclasts is completely filled with new osteoid material secreted by active osteoblasts during a reversal phase. The mesenchymal stem cells are attracted to BMU sites and differentiated into osteoblasts. The active osteoblasts secrete osteocalcin (OC), type-I pro-collagen peptides (N-terminal-PINP and C-terminal-PICP), and bone specific alkaline phosphatase (skeletal ALP); the concentration of OC, P1NP, P1CP, and skeletal ALP in serum is correlated with the bone formation rate.[16] Bone formation is completed by mineralization, which occurs in two phases. The first phase occurs immediately after the formation of osteoid, when hydroxyapatite crystals are deposited between organic matrixes. The second phase involves a slower 'secondary mineralization' process that continues over months when more mineral is gradually added [Figure 1(C-D)]. Secondary mineralization increases the BMD, but not the volume, of new bone.[22]
Figure 1.
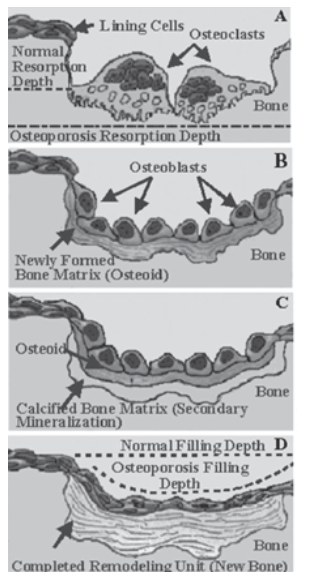
Bone remodeling cycle and bone biochemical markers. The bone remodeling cycle is initiated by osteoclasts, which create resorption pits with low pH microenvironment (A), dissolves the inorganic matrix exposing the organic matrix (> 90% of the organic matrix is composed of type-I collagen). Then bone-reabsorbing enzymes digest the organic bone matrix releasing breakdown products of type-I collagen. The collagen breakdown products include terminal peptide fragments from both ends of the type-I collagen such as (N-terminal telopeptides - NTx, and C-terminal telopeptides - CTx) and a ring structure called deoxypyridinoline (DPD). Blood and urine levels of these collagen breakdown products indicate bone resorption rate. The normal bone turnover process creates resorption cavities, which are smoothed off by osteoblasts (B). The active osteoblasts secrete osteocalcin (OC), type- I pro-collagen peptides (N-terminal-PINP and C-terminal-PICP), and bone specific alkaline phosphatase (skeletal ALP); the concentration of OC, P1NP, P1CP, and skeletal ALP in serum is a measure of bone formation rate.[16] Bone formation is completed by mineralization, which occurs in two phases. The first phase occurs immediately after the formation of osteoid, when hydroxyapatite crystals are deposited between organic matrixes. The second phase involves a slower 'secondary mineralization' process that continues over months when more mineral is gradually added (D).
Bone formation and bone resorption are usually balanced in healthy individuals who have adequate nutrition and exercise and attain normal puberty. Bone mass peaks at 30 years in both men and women and approximately 0.4% of bone is lost per year after this peak bone mass is achieved. In addition to this loss, approximately 1%-2% of bone is lost per year for the first 5-8 years after menopause.[18] With menopause and aging, a coordinated balance in bone formation and resorption is disturbed, resulting in excessive bone loss and osteoporosis. The main factors that play important roles in pathogenesis of osteoporosis include increased: (1) frequency of BMU activation by up-regulation of osteoclasts; and (2) activity of osteoclasts. In addition, suppressed activity of osteoblasts and inadequate bone formation are the main causes of progression, thus providing opportunity for new therapeutic approaches to stimulate bone formation. In osteoporosis patients, how increased bone remodeling affects loss in bone strength is shown in Figure 1(A-D), the osteoclasts create more and excessively deep cavities (Figure 2B) resulting in: (1) increased porosity and significantly reduced bone strength in cortical bone; and (2) thinning of trabeculae and loss of trabecular connectivity in cancellous bone, reducing the bone strength by many fold. Excessive bone resorption creates small areas of clustered deep cavities that act as stress concentrators,[23,24] which is analogous to a notch drawn (by a diamond cutter) on a glass plate to initiate a crack along a cutting line. Consequently, the stress concentrators represent a focal weakness, which signifycantly decreases the stress required to initiate fracture (shown in Figure 2). In addition, as horizontal trabeculae, which act as crossties slowly disappear due to increased remodeling, the thin unsupported vertical trabeculae are predisposed to buckling pressure. This loss of connectivity of horizontal trabeculae has a major impact on fracture risk independent of other factors affecting bone quality.[25,26] Thus far, no direct evidence is available that directly relates bone marker levels with bone strength, however, high levels of bone resorption markers correlate with high rates of bone remodeling and therefore, theoretically bone markers can independently predict fracture risk. This concept also illustrates that small local losses of bone may not decrease much of the bone density but such losses can markedly decrease bone strength and thereby increase the risk for bone fracture.
Figure 2.
Small changes in bone turnover make large changes in bone strength. (A) Cross-section of a vertebral body from a normal person showing the pattern of spinal trabecular bone, and (B) that of a patient with osteoporosis showing the 'thinning' and loss of spinal trabecular bone, which compromises the overall mechanical strength of the bone. On the right hand panels, the illustrations show how high bone turnover affects trabecular bone micro-architecture and causes fractures. The normal bone turnover process creates resorption cavities, which are smoothed off by osteoblasts (A). In osteoporosis patients, the osteoclasts create more and excessively deep cavities (B) causing loss of trabecular connectivity, which is independently associated with fracture risk.[25,26] In addition, small areas of clustered deep cavities on a thin unsupported vertical trabeculae act as stress concentrators,[23,24] which significantly decrease the stress required for fracture (as shown in the example of a walking stick). This concept also illustrates that small local losses of bone may not decrease much of the bone density but markedly decrease the bone strength.
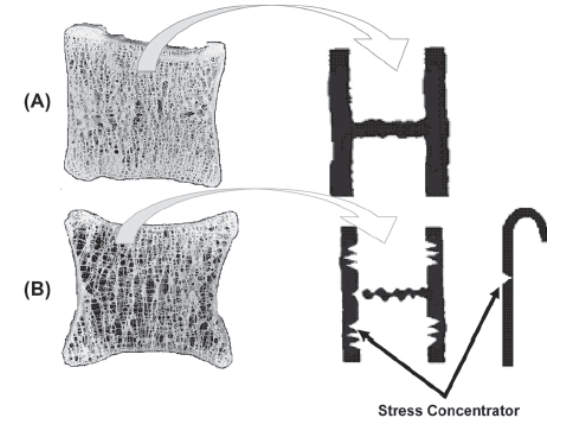
It is important to note that because bone resorption and formation are coupled, an increase in either process will increase bone marker levels. In chronic steady-state conditions, most markers reflect both formation and resorption because only a small imbalance exists. In addition, biochemical markers are not disease specific, but reflect alterations in skeletal metabolism regardless of the underlying cause.
There are several reasons for familiarizing the primary care physicians with the role bone markers can play in the management of osteoporosis at this point in time:
1. Biochemical markers could be useful in making therapeutic decisions because, in general, the higher the bone remodeling and bone loss rates[27,28] the greater the concentration of bone biochemical markers in blood or urine. This relationship appears to be continuous, with increasing levels of bone biochemical markers associated with progressively greater risk of rapid bone loss. Women generally lose about 1%-2% of their bone per year during and after menopause. However, nearly a third of postmenopausal women lose bone at a faster rate (3%-4% per year).[27-29] Bone biochemical markers may detect these patients who are considered 'high bone turnover' patients who, are also most likely to respond to an osteoporosis therapy.[28,29] A high turnover rate is detrimental to bone quality as explained above. In addition, a high bone turnover rate can reduce bone strength because old bone that has undergone more complete secondary mineralization is replaced by younger bone that has undergone primary, but less complete secondary, mineralization.[12] The foregoing suggest that a higher rate of excretion of bone biochemical markers indicates a high bone turnover state, which is detrimental to bone strength thus bone markers may be potentially useful in identifying patients with increased risk of fracture.[30]
2. At present BMD measurement is the best surrogate clinical marker of fracture risk, however, change in BMD during therapy is a poor predictor of fracture risk.[7,8,10,11] The reduction in fracture risk explained by the observed changes in BMD by alendronate (6%-7% increase in BMD in the Fracture Intervention Trial),[31] residronate (5%-6% increase in BMD in the Vertebral Efficacy With Residronate Therapy Study),[32] raloxifene (2% increase in BMD in the Multiple Outcome of Raloxifene Evaluation study),[33] and calcitonin (1.2% increase in BMD in the PROOF study)[34] therapy is approximately 16%, 4%, 28%, and 8% respectively, indicating other factors contribute to bone strength and change with treatment. Several recent studies have shown[13,14,35,36] that decreases in bone biochemical markers can account for 40%-70% of the observed anti-fracture efficacy. Data are beginning to accrue suggesting that changes in bone biochemical markers during treatment of osteoporosis patients can predict subsequent reductions in fracture risk[13,14] independent of bone density by mechanisms that explain improvement in bone strength.[37]
3. In the management of osteoporosis, changes in bone density generally can only be seen, on average, following about 2 years of treatment, whereas biochemical markers can provide dynamic information on the effectiveness of therapeutic agents within 3-6 months.[38, 39] Importantly, currently available osteoporosis therapies such as bisphosphonates, estrogens, SERMS, and calcitonins, act by reducing bone biochemical markers, thereby reducing the bone marker levels; hence, markers are useful in assessing efficacy of therapy.
4. Since November 2002, Medicare reimburses for several bone marker tests for both men and women.[40]
In this article we review the potential utility of bone markers with respect to four general topics. (1) Why use bone markers in clinical practice? (2) Who should be tested? (3) How does one use bone markers in the therapeutic management of osteoporosis? (4) The use of a clinical algorithm regarding use of bone markers in the management of osteoporosis. As we discuss the value of bone markers in the management of osteoporosis, it should be noted that none of the markers are diagnostic for any particular bone disease nor are markers substitutes for BMD measurement. Furthermore, there are no prospective studies showing that the use of markers improves outcomes, such as fracture rate, morbidity, or mortality. Nevertheless, there is evidence that markers provide different information than bone density and their measurement can complement BMD to individualize patient management.
Why Use Bone Markers in Clinical Practice?
Bone Markers Can Identify Patients With Risk of Rapid Bone Loss
Bone mineral density in osteoporotic patients results from both peak bone density and subsequent postmenopausalor age-related bone loss. Longitudinal studies of postmenopausal women[28-30] have demonstrated two characteristic groups: those losing a significant amount of bone mineral (the 'high bone turnover' group), and those losing a normal amount of bone mineral (the 'normal or low bone turnover' group). Patients with 'high bone turnover' appear to have higher levels of bone biochemical markers in blood and urine. Several studies indicate that, at least for groups of individuals, bone biochemical markers can be used to estimate the rate of bone loss. Longitudinal studies of cohorts that have not undergone treatment for postmenopausal osteoporosis suggest that baseline bone marker levels are associated with those who will lose bone density most rapidly. Figure 3 shows how baseline levels of a urinary resorption marker, NTx, are associated with subsequent rapid bone loss measured after 1 year. A high baseline NTx, > 67 units, indicated a 17.3 times higher risk of BMD loss if not treated.[22] Similarly, a 4- year follow-up study of postmenopausal women[35] found that women with normal bone biochemical markers lost less than 1% BMD over 4 years, while those with higher levels of bone biochemical markers lost 3-5 times higher amount of bone. Therefore, biochemical bone markers may be used for identifying those subjects that are losing bone most rapidly. This is particularly important, because patients with rapid bone loss will show the greatest stabilizations and increases in bone density in response to anti-resorptive medications.[17]
Figure 3.
Bone markers predict rapid bone loss. Baseline data on N-terminal telopeptides of type-I collagen (NTx, expressed as a ratio of creatinine), in a randomized placebo-controlled trial in younger postmenopausal women[27] predicts loss in lumbar spine BMD (L1-L4) after 1 year. The authors averaged four NTx values at baseline and during the course of study found that subjects with highest NTx values experienced the greatest spinal BMD loss (the high baseline NTx, greater than 67 nM BCE/nM creatinine, indicated a 17.3 times higher risk of BMD loss). (NTx = collagen type I cross-linked amino terminal peptide)
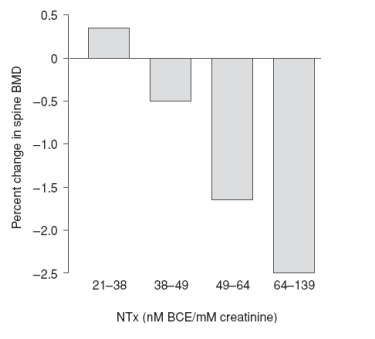
In summary, the 'high turnover group' appears to have elevated concentrations of biochemical markers compared to the 'normal turnover group', consequently, measurement of bone biochemical markers may have a potential future role in the identification and management of the patient with osteoporosis. However, clinical utility of biochemical bone markers to classify individual patients is not yet fully established. For example, recent data from the Fracture Intervention Trial[14] and OFELY study[41] showed that 20%-30% of patients classified as 'high bone turnover' on the basis of bone biochemical markers at baseline could be classified inaccurately, possibly due to biological variation. In addition, it should be noted that elevated markers do not always indicate bone loss; important exceptions include growth period during early life or during treatment with parathyroid hormone (PTH) where accelerated formation exceeds resorption and thus, increased bone marker levels indicate bone gain.[42,43]
High Rate of Bone Turnover is Associated With Increased Risk of Fracture
There is evidence that high bone turnover, as measured by bone markers, is associated with increased fracture risk. The relationship between biochemical markers and fracture risk has been investigated in retrospective studies[36,44] comparing markers in patients with osteoporotic fractures and in controls, and in prospective studies,[30, 36] where markers were measured before the occurrence of fractures. In a prospective study[30] involving 7598 healthy women of more than 75 years of age, the authors demonstrated that increased rates of bone turnover might identify those patients at highest risk for hip fracture, indicated by increased excretion of the urinary resorption marker CTx with an odds ratio of 2.2 (Figure 4). Women with both low femoral neck bone mineral density and increased urinary CTx levels were at greater risk of hip fracture with an odds ratio of 4.8. In another example of how bone markers are associated with increased fracture risk, Hochberg and co-workers[36] performed a meta-analysis of 18 clinical trials of anti-resorptive therapy, which showed a 70% reduction in resorption markers would be associated with a 40% reduction in non-vertebral fractures, and a 50% reduction in formation markers would be associated with a 44% reduction in fracture risk.
Figure 4. Bone markers predict fracture risk. In a prospective study by Garnero et al,[30] involving 7598 elderly women, increased excretion of urinary C-telopeptide of type-I collagen (CTx) was associated with increased risk of hip fracture with an odd ratio of 2.2, independent of bone mineral density (BMD). The combination of low hip BMD and increased excretion of resorption marker CTx enhances hip fracture prediction with an odds ratio of 4.8. (CTx = collagen type I crosslinked carboxyl terminal peptide)
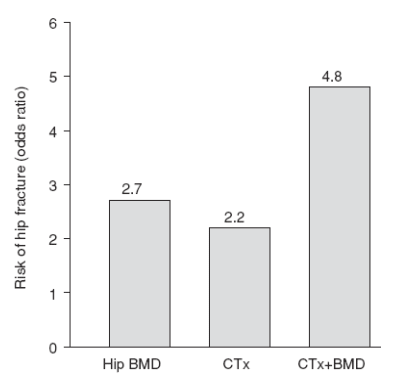
Despite this promising evidence, the ability of markers to predict fractures has not been fully established; thus, bone markers are neither substitutes nor surrogates for BMD measurements to predict fractures. Additional studies involving bone biochemical markers as primary outcome variables are required to confirm some of these findings.
Bone Markers Predict Response to Therapy Before Evidence of BMD Changes
Measurements of bone density are comparatively less effective in terms of measuring acute changes in bone because they represent a net balance between bone resorption and bone formation, which typically changes in response to therapy less than 2% per year or a maximum of < 1% in 3-6 months. However, changes of < 1% are difficult to measure due to precision limitations of BMD technologies.[45] In contrast, osteoporosis therapies such as hormone replacement,[27] SERM's,[12] bisphosphonates,[13,35,38] and calcitonin[46,47] act by reducing the bone marker levels by 30%-70% within 3-6 months ( Table 2 ). Even though bone density measurements are much more precise than biochemical bone markers, it is still easier to detect a 50% change with a biochemical bone marker than a 1% change in BMD with a bone densitometer after 3-6 months of therapy (Figure 5). The rapid changes in bone markers may allow making important therapeutic decisions for optimal management of osteoporosis patients, particularly if osteoporosis is severe.
Figure 5. Changes in BMD and bone markers in response to bisphosphonate therapy of osteoporosis patients. A precision error of 1%-1.5% in BMD measurements means that 2 years of treatment may be needed between testing to observe a significant change in BMD. However, a 50% change in bone resorption marker after only 3 months may mean a significant change in response to treatment. The shaded area shows minimum change required in some urinary resorption markers (decrease) and in BMD (increase) to be considered a true biologic change. Only changes greater than the LSC indicate treatment-related effects. The illustration shows average changes in BMD and markers[13,14,35,36,38] in response to treatment with a bisphosphonate; data is taken from the studies listed in Table 2
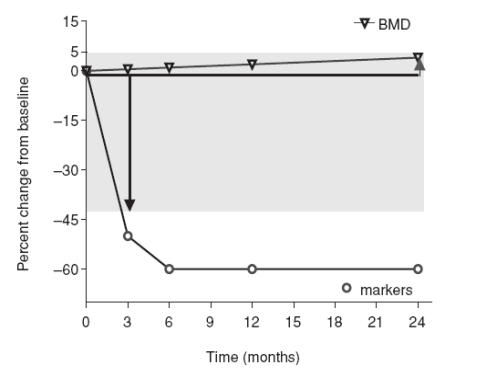
In the clinical management of osteoporosis patients, noncompliance with medication is a significant issue, as seen for a number of chronic medical conditions.[48] Studies have found up to 50% of patients started on osteoporosis medications are no longer taking them 1 year after they are prescribed.[49-51] Preliminary evidence reported from some recent studies indicates that use of frequent physician visits[53] and biochemical marker tests[54,55] that confirm treatment efficacy may increase medication compliance. However, results of these studies did not demonstrate that monitoring with markers results in higher BMD or fewer fractures among treated women.
Who Should Be Tested?
The aim of bone marker analysis is to individualize treatment and the consensus of experts in this area is that measurement of bone turnover markers provides potentially useful information to supplement BMD measurement.[16] Currently, the only way to determine fracture risk for osteoporosis is to have a BMD measurement. Guidelines on when and whom to screen for BMD have been developed by various professional organizations and independent studies,[56-61] many of which recommend BMD screening in women at risk for osteoporosis. The National Osteoporosis Foundation (NOF),[59] the US Preventive Services Task Force (USPSTF),[60] and the International Society for Clinical Densitometry (ISCD)[61] recommend conditions under which BMD screening is appropriate for women. ISCD guidelines also address BMD testing in men. Once a BMD has been obtained, we suggest that all patients with severe osteoporosis (bone density less than -2.5 T-score) should be tested for biochemical bone markers so that patients with high bone markers can be identified and evaluated for necessary changes in dose or type of therapeutic agents that could be made in an effort to prevent further bone loss and reduce fracture risk. For patients with moderately low BMD (T-Score < -1.0 and > -2.5), who have been recently shown to have a large number of fractures in the National Osteoporosis Risk Assessment (NORA) study,[63] we suggest that these patients be evaluated for other clinical risk factors associated with fractures, such as: (1) previous low trauma fractures (above age 45 years); (2) current cigarette smoking; (3) steroid exposure anytime; (4) family history of hip fractures; (5) high alcohol intake (> 2 units/day); (6) rheumatoid arthritis; (7) increase in falls or frailty. Patients who have moderately low bone density (T-Score < -1.0 and > -2.5) and one or more of the above risk factors should be tested for bone markers for further follow-up. Furthermore, the Medicare National Coverage Decision (NCD) lists 22 diagnoses[40] or conditions for which bone markers such as collagen crosslink tests could be reasonable.
How Does One Use Bone Markers in the Therapeutic Management of Osteoporosis?
How Does Variability Affect Application of Bone Markers in Clinical Practice?
In the past, practical utilization of markers was limited by variability in bone markers, which was considered too great for diagnostic use in individual patients. New assays are more specific and we know how to apply them more intelligently by controlling the pre-analytical and analytical sources of variability,[38,63-66] such as food intake (fasting blood sample) and time of sample collection can reduce variability of CTx test to 14%, as compared to 34% for non-fasting samples.[67] To minimize the analytical variance most of the bone marker tests are now performed on fully automated clinical analyzers. For monitoring response to treatment in individual patients, use of least significant change[38] (LSC) has been suggested. The LSC defines a true biological change due to therapeutic intervention in a patient taking into account total variation (biological and analytical) in a marker. LSC can be calculated by the following formula: LSC = 1.96 X √ 2 X √(CVa)2 + (CVi)2. Where CVa is analytical error and CVi is long term within-individual variation. The LSC values for most of the bone markers, listed in Table 1 , range between 15% and 40%. Additional measures suggested to reduce variability is collection and measurement of dual samples, which will further reduce the biological variance and hence LSC [under these condition LSC = 1.96 X √(CVa)2 + (CVi)2).[65]
As shown in Figure 5, for most bone biochemical markers the LSC is approximately 30%-40% as compared to LSC of 4%-5% for changes in BMD (LSC is shown as the shaded area in Figure 1). Only changes greater than the LSC indicate treatment-related effects. The currently approved anti-resorptive therapies induce reductions of 50%-70% in collagen crosslink markers as compared to baseline values. This implies that bone markers can be used to monitor therapy in spite of this large variation. For example, to monitor therapy, a post-treatment value in bone marker is compared with baseline values determined before initiating therapy. If the percentage change in the post-treatment value is greater than LSC (most markers show 40%-70% changes in less than 3 months as shown as in Table 2 and Figure 5) then it is considered a true biological change due to therapy and not due to biological variation in bone marker in an individual.
At What Time-Point Should a Bone Marker Measurement Be Performed?
A baseline value, by collecting two consecutive samples (collected anytime between 1 and 12 weeks), whenever possible, must be obtained for all patients where bone markers are intended to follow up the bone turnover. Due to reduced biological variance and LSC, a bone marker value generated from the mean of two measurements will provide a more reliable estimate of baseline value for further follow-up.[14,38,65] For patients on alendronate, residronate or raloxifene,[38,39] bone marker testing is repeated at 3 months. For patients on HRT,[27,28] bone marker measurements are repeated at 6 months. Additional follow-up of one bone marker measurement per year is permitted by Medicare after 12 months and thereafter.[40]
How to Classify Patients With 'High Bone Turnover' or 'High Risk'
A patient's pretreatment bone biochemical marker status is defined as 'low risk' or 'high risk' (for further follow-up) by comparing their pretreatment bone marker value with a mean value derived from normative reference population, as shown in Table 3 . For example, for monitoring postmenopausal women, the premenopausal range + 1SD (as shown in Figure 6) is usually used because it corresponds to the upper limit of estrogen-complete women who have stable bone turnover and do not lose bone. The rationale behind this approach is based on popular use of standard deviation as cut-off to determine the relationship between bone biochemical marker levels in blood and urine and longterm loss in bone density and/or increase in fracture risk in postmenopausal women in most of the clinical trials. Other methods of stratification involve use of tertiles or quartiles. In summary, there is no consensus by which to define 'low risk' and 'high risk' groups, but an upper limit of the reference ranges seems clinically most relevant.
Figure 6. A suggested algorithm for the use of biochemical markers of bone turnover in making therapeutic decisions on treatment and follow-up of postmenopausal patients with osteopenia and osteoporosis
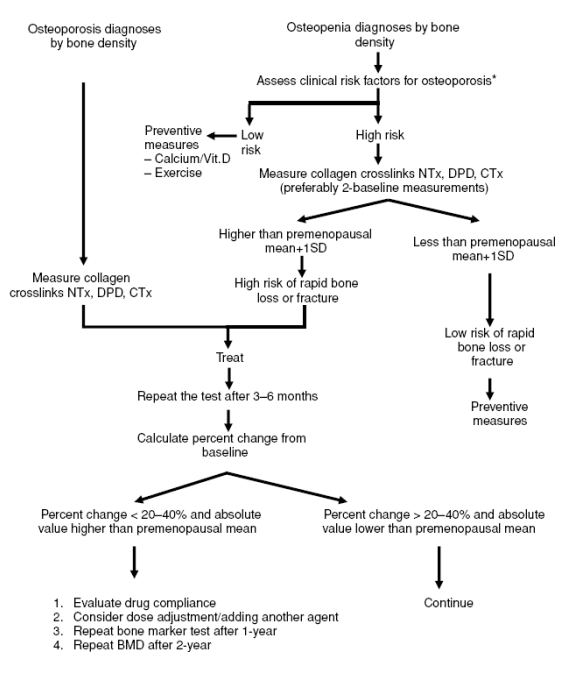
Reimbursement of Bone Marker Tests
The Centers for Medicare and Medicaid Services (CMS) has established a national policy for reimbursement of collagen crosslink testing, as of November 25, 2002.[40] The Medicare NCD for urine based collagen crosslink tests (CPT 82523) lists three uses under 'Indications':
identify individuals with elevated bone resorption, who have osteoporosis in whom response to treatment is being monitored;
predict response (as assessed by bone mass measurements) to FDA approved anti-resorptive therapy in postmenopausal women;
assess response to treatment of patients with osteoporosis, Paget's disease of the bone, or risk of osteoporosis where treatment may include FDA approved anti-resorptive agents, anti-estrogens or selective estrogen receptor moderators.
A Clinical Algorithm to Use Bone Markers in Treatment and Management of Osteoporosis
The best use of bone markers is in classifying patients with low or moderately low bone density into 'high risk' and 'low risk' categories based on bone marker measurements. Consequently, we suggest a clinical algorithm (Figure 6) based on an analogous algorithm published for BMD[68] and bone markers[69] to individualize therapy and reduce fracture risk. In addition, when a patient is taking his/her medications but bone density is not improving, or fracture occurs, bone marker tests can be done to see if addition of further medications might be helpful. The best management of patients with osteoporosis that have a low bone turnover is not clear at this time. Biochemical marker data suggest that these patients would be the least likely to respond favorably to anti-resorptive therapy. However, this issue needs to be investigated further. Such patients could be eligible for treatment with PTH 1-34,[70] an anabolic agent.
CONCLUSION
The best use of bone markers is in classifying patients with low or moderately low bone density into 'high risk' and 'low risk' categories based on bone marker measurements. Consequently, we suggest a clinical algorithm (Figure 6) based on an analogous algorithm published for BMD[68] and bone markers[69] to individualize therapy and reduce fracture risk. In addition, when a patient is taking his/her medications but bone density is not improving, or fracture occurs, bone marker tests can be done to see if addition of further medications might be helpful. The best management of patients with osteoporosis that have a low bone turnover is not clear at this time. Biochemical marker data suggest that these patients would be the least likely to respond favorably to anti-resorptive therapy. However, this issue needs to be investigated further. Such patients could be eligible for treatment with PTH 1-34,[70] an anabolic agent.
REFERENCES
1. National Osteoporosis Foundation. Osteoporosis: review of the evidence for prevention, diagnosis, and treatment and cost effective analysis. Osteoporosis Int 1998;8:S1-S88
2. NIH Consensus Development Panel on Osteoporosis. J Am Med Assoc 2001:285;785-95
3. Consensus Development Conference. Diagnosis, prophylaxis and treatment of osteoporosis. Am J Med 1993;94:646-50
4. WHO Study Group. Assessment of fracture risk and its application to screening for postmenopausal osteoporosis: report of a WHO Study Group. WHO Technical Report Series 843, Geneva, Switzerland: World Health Organization; 1994. p. 1-129
5. Cummings SR, Black DM, Nevitt MC, et al. Bone density at various sites for prediction of hip fractures [The Study of Osteoporotic Fractures Research Group]. Lancet 1993;341:72-5
6. Burr DB. Introduction - bone turnover and fracture risk. J Musculoskeletal Neuron Interact 2003;3(4):408-9
7. Cummings SR, Karpf DB, Harris F, et al. Improvement in spine bonedensity and reduction in risk of vertebral fractures during treatment with antiresorptive drugs. Am J Med 2002;112:281-9
8. Sarkar S, Mitlak BH, Wong M, Stock J, Black DM, Harper KD. Relationships between bone mineral density and incident vertebral fracture risk with raloxifene therapy. J Bone Miner Res 2002;17:1-10
9. Heaney RP. Is the paradigm shifting? Bone 2003;3(4):457-65
10. Riggs BL, Melton III LJ. Bone turnover matters: the raloxifene treatment paradox of dramatic decrease in vertebral fractures without commensurate increase in bone density. J Bone Miner Res 2002;17:11-4
11. Melton LJ, Khosla S, Atkinson EJ, O'Fallon WO, Riggs BL. Relationship of bone turnover to bone density and fractures. J Bone Miner Res 1997;12:1083-91
12. Dempster DW. The impact of bone turnover and boneactive agents on bone quality: focus on hip. Osteoporosis Int 2002;13:349-52
13. Sarkar S, Reginster J, Crans GG, Diez-Perez A, Pinette Karen V, Delmas PD. Relationship between changes in biochemical markers of bone turnover and BMD to predict vertebral fracture risk. J Bone Miner Res 2004;19(3):394-401
14. Bauer, DC, Black DM, Garnero, P, et al., for the Fracture Intervention Trial Study Group. Change in bone turnover and hip, non-spine, and vertebral fracture in alendronate-treated women: the fracture intervention trial. J Bone Miner Res 2004;19(8):1250-8
15. Parfitt AM. High bone turnover is intrinsically harmful: two paths to a similar conclusion. The Parfitt view. J Bone Miner Res 2002;17(8):1558-9 [discussion 1560]
16. Miller PD, Baran DT, Bilezekian J, et al. Practical clinical applications of biochemical markers of bone turnover. J Clin Densitometry 1999;2(3):323-42
17. Agerbaek MO, Eriksen EF, Kragstrup J, Mosekilde L, Melsen F. A reconstruction of the remodeling cycle in normal human cortical iliac bone. Bone Miner 1991;12(2):101-12
18. Manolagas SC. Birth and death of bone cells: basic regulatory mechanisms and implications for the pathogenesis and treatment of osteoporosis. Endocr Rev 2000;21:115-37
19. Hanson DA, Weis MA, Bollen AM, Maslan SL, Singer FR, Eyre DR. A specific immunoassay for monitoring human bone resorption: quantitation of type I collagen cross-linked Ntelopeptides in urine. J Bone Miner Res 1992;7(11):1251-8
20. Bonde M, Qvist P, Fledelius C, Riis BJ, Christiansen C. Immunoassay for quantifying type I collagen degradation products in urine evaluated. Clin Chem 1994;40(11):2022-5
21. Bettica P, Taylor AK, Talbot J, Talamani R, Baylink DJ. Clinical performance of galactosyl hydroxylysine, pyridinoline, and deoxypyridinoline in postmenopausal osteoporosis. J Clin Endocrinol Metab 1996;81:542-6
22. Boivin G, Meunier PJ. The degree of mineralization of bone tissue measured by computerized quantitative contact microradiography. Calcif Tissue Int 2002;70(6):3-11
23. Einhorn TA. Bone strength: the bottom line. Calcif Tissue Int 51;1992:333-9
24. Parfitt AM. Use of bisphosphonates in the prevention of bone loss and fractures. Am J Med 1991;91(5B):42S-46S
25. Oleksik A, Ott SM, Vedi S, Bravenboer N, Compston J, Lips P. Bone structure in patients with low bone mineral density with or without vertebral fractures. J Bone Miner Res 2000;15(7): 1368-75
26. Legrand E, Chappard D, Pascaretti C, et al. Trabecular bone microarchitecture, bone mineral density, and vertebral fractures in male osteoporosis. J Bone Miner Res 2000;15(1):13-9
27. Chestnut CH, Clark GS, English SC, et al. Hormone replacement therapy in postmenopausal women: urinary N-telopeptide of type-I collagen monitors therapeutic effect and predicts response of bone mineral density. Am J Med 1997;102:29-37
28. Ross PD, Knowlton W. Rapid bone loss is associated with increased levels of biochemical markers. J Bone Miner Res 1998;13(2):297-302
29. Garnero P, Sornay-Rendu E, Duboeuf F, Delmas PD. Markers of bone turnover predict postmenopausal forearm bone loss over 4 years: the OFELY study. J Bone Miner Res 1999;14(9): 1614-21
30. Garnero P, Hausherr E, Chapuy MC, et al. Markers of bone resorption predict hip fracture in elderly women: the EPIDOS Prospective Study. J Bone Miner Res 1996;11(10):1531-8
31. Black DM, Thompson DE, Bauer DC, et al. Fracture risk reduction with alendronate in women with osteoporosis: the Fracture Intervention Trial [FIT Research Group]. J Clin Endocrinol Metab 2000;85(11):4118-24
32. Reginster JY, Minne HW, Sorenson OH, et al. Randomized trial of the effects of residronate on vertebral and non-vertebral fractures in women with postmenopausal osteoporosis: a randomized controlled trial [Vertebral Efficacy With Residronate Therapy (VERT) Study Group]. J Am Med Assoc 1999;282(14): 1344-52
33. Ettinger B, Black DM, Mitlak BH, et al. Reduction of vertebral fracture risk in postmenopausal women with osteoporosis treated with raloxifene: results from a 3-year randomized clinical trial. J Am Med Assoc 1999;282:637-45
34. Chesnut 3rd CH, Silverman S, Andriano K, et al. A randomized trial of nasal spray salmon calcitonin in postmenopausal women with established osteoporosis: the prevent recurrence of osteoporotic fractures study [PROOF Study Group]. Am J Med 2000;109(4):267-76
35. Eastell R, Barton I, Hannon RA, Chines A, Garnero P, Delmas PD. Relationship of early changes in bone resorption to the reduction in fracture risk with risedronate. J Bone Miner Res 2003;18(6):1051-6
36. Hochberg MC, Greenspan S, Wasnich RD, Miller P, Thompson DE, Ross PD. Changes in bone density and turnover explain the reductions in incidence of non-vertebral fractures that occur during treatment with antiresorptive agents. J Clin Endocrinol Metab 2002;87(4):1586-92
37. Dobing H, Fahrleitner A, Ste-Marie L, et al. Early changes in biochemical markers of bone formation predict improvements in bone structure during teriparatide therapy. 85th Annual Meeting of The Endocrine Society, Program & Abstracts, 2003. p. 131
38. Braga de Castro-Machado A, Hannon R, Eastell R. Monitoring alendronate therapy for osteoporosis. J Bone Miner Res 1999;14(4):602-8
39. Raisz L, Smith JA, Trahiotis M, et al. Short-term risedronate treatment in postmenopausal women: effects on biochemical markers of bone turnover. Osteoporos Int 2000;11(7):615-20
40. Medicare program; negotiated rulemaking: coverage and administrative policies for clinical diagnostic laboratory services; final rule. November 23, 2001, [66 FR 58843-58846]
41. Garnero P, Mulleman D, Munoz F, Sornay-Rendu E, Delmas PD. Long-term variability of markers of bone turnover in postmenopausal women and implications for their clinical use: the OFELY study. J Bone Miner Res 2003;18(10):1789-94
42. Lane NE, Sanchez S, Genant HK, Jenkins DK, Arnaud CD. Short-term increases in bone turnover markers predict parathyroid hormone-induced spinal bone mineral density gains in postmenopausal women with glucocorticoid-induced osteoporosis. Osteoporosis Int 2000;11(5):434-42
43. Burr DB, Hirano T, Turner CH, Hotchkiss C, Brommage R, Hock JM. Intermittently administered human parathyroid hormone (1-34) treatment increases intracortical bone turnover and porosity without reducing bone strength in the humerus of ovariectomized cynomolgus monkeys. J Bone Miner Res 2001;16(1):157-65
44. Akesson K, Ljunghall S, Jonsson B, et al. Assessment of biochemical markers of bone metabolism in relation to the occurrence of fracture: a retrospective and prospective population-based study of women. J Bone Miner Res 1995;10(11):1823-9
45. Ravaud P, Reny JL, Giraudeau B, Porcher R, Dougados M, Roux C. Individual smallest detectable difference in bone mineral density measurements. J Bone Miner Res 1999;4(8):1449-56
46. Trovas GP, Lyritis GP, Galanos A, Raptou P, Constantelou EA. Randomized trial of nasal spray salmon calcitonin in men with idiopathic osteoporosis: effects on bone mineral density and bone markers. J Bone Miner Res 2002;17(3):521-7
47. Srivastava AK, Libanati C, Hohmann O, Kriegman A, J Baylink DJ. Effects of calcitonin nasal spray on serum C-telopeptide levels in elderly osteopenic women with increased bone turnover. Calcif Tissue Int 2004;75:477-81
48. Haynes RB, Montague P, Oliver T, McKibbon KA, Brouwers MC, Kanani R. Interventions for helping patients to follow prescriptions for medications. Cochrane Database Syst Rev CD000011, 2000
49. Turbi C, Herrero-Beaumont G, Acebes JC, et al. Compliance and satisfaction with raloxifene versus alendronate for the treatment of postmenopausal osteoporosis in clinical practice: an open-label, prospective, nonrandomized, observational study. Clin Ther 2004;26(2):245-56
50. Davies GC, Huster WJ, Lu Y, Plouffe Jr L, Lakshmanan M. Adverse events reported by postmenopausal women in controlled trials with raloxifene. Obstet Gynecol 1999;93:558-65
51. Recker RR, Gallagher R, Amonkar M, Smith JC, MacCosbe PE. Medication persistence is better with weekly bisphosphonates, but it remains suboptimal. Poster SA407, presented at: 26th Annual Meeting of the American Society for Bone Mineral Research, October 1-5, 2004, Seattle, WA
52. Clowes JA, Peel NFA, Eastell R. The effect of monitoring on adherence and persistence with raloxifene therapy and the impact of the effectiveness of treatment. J Bone Miner Res 2002;17(Suppl 1):S155
53. Clowes JA, Peel NF, Eastell R. The impact of monitoring on adherence and persistence with antiresorptive treatment for postmenopausal osteoporosis: a randomized controlled trial. J Clin Endocrinol Metab 2004;89(3):1117-23
54. Eastell R, Garnero P, Vrijens B, et al. Influence of patient compliance with risedronate therapy on bone turnover marker and bone mineral density response: the IMPACT study. Presented at the 30th European Symposium on Calcified Tissues, May 8-12, 2003, Rome, Italy
55. Delmas PD, Vrijens B, Roux C, et al. A reinforcement message based on bone turnover marker response influences long-term persistence with risedronate in osteoporosis: the IMPACT study. J Bone Miner Res. 2003;18(Suppl 2):S374
56. The Swedish Council on Technology Assessment in Health Care. Bone density measurement - a systematic review: a report from SBU. J Intern Med 1997;241(Suppl 739):5-11,12-22,42-4
57. Assessment of osteoporotic fracture risk and its application to screening for postmenopausal osteoporosis. Report of a WHO Study Group. World Health Organ Tech Rep Ser 1994;843:1-129
58. Osteoporosis prevention, diagnosis, and therapy. NIH Consens Statement 2000;17(1):1-36
59. Physician's Guide to Prevention and Treatment of Osteoporosis. 2nd ed. Washington, DC: National Osteoporosis Foundation; Position statement: executive summary
60. Unites States Preventive Services Task Force. Screening for osteoporosis in postmenopausal women: recommendations and rationale. Ann Intern Med 2002;137(6):526-8. Source description. Available from www.ahrq.gov/clinic/epcsums/ osteosum.htm
61. The Writing Group for the International Society for Clinical Densitometry (ISCD) Position Development Conference. J Clin Densitom. 2004;7:7-12
62. Siris ES, Miller PD, Barrett-Connor E, Faulkner KG, Wehren LE, Abbott TA, Berger ML, Santora AC, Sherwood LM. Identification and fracture outcomes of undiagnosed low bone mineral density in postmenopausal women: results from the National Osteoporosis Risk Assessment. JAMA. 2001;286(22):2815-22
63. Hannon R, Blumsohn A, Naylor K, Eastell R. Response of biochemical markers of bone turnover to hormone replacement therapy: impact of biological variability. J Bone Miner Res 1998;13(7):1124-33
64. Eastell R, Mallinak N, Weiss S, Ettinger M, Pettinger M, Cain D, et al. Biological variability of serum and urinary N-telopeptides of type I collagen in postmenopausal women. J Bone Miner Res 2000;15(3):594-8
65. Hannon R, Eastell R. Preanalytical variability of biochemical markers of bone turnover. Osteoporosis Int 2000;11 Suppl 6: S30-44
66. Vesper HW, Demers LM, Eastell R, Garnero P, Kleerekoper M, Robins SP, et al. Assessment and recommendations on factors contributing to preanalytical variability of urinary pyridinoline and deoxypyridinoline. Clin Chem 2002;48(2):220-35
67. Qvist P, Christgau S, Pedersen BJ, Schlemmer A, Christiansen C. Circadian variation in the serum concentration of C-terminal telopeptide of type I collagen (serum CTx): effects of gender, age, menopausal status, posture, daylight, serum cortisol, and fasting. Bone. 2002:31(1);57-61
68. South-Paul JE. Osteoporosis Part I. Evaluation and Assessment. Am Family Phy 2001:63(5);897-904
69. Nishizawa Y, Nakamura T, Ohata H, et al. Guidelines on the use of biochemical markers of bone turnover in osteoporosis (2001). J Bone & Miner Metabolism 2001;19:338-44
70. Neer RM, Arnaud CD, Zanchetta JR, et al. Effect of parathyroid hormone (1-34) on fractures and bone mineral density in postmenopausal women with osteoporosis. N Engl J Med. 2001;344:1434-41
|
|
|
|
|
|
|