|
New Therapies for Hepatitis C Virus Infection: REVIEW ARTICLE
|
|
|
New Therapies for Hepatitis C Virus Infection in PDF
Clinical Infectious Diseases Feb 12009;48:313-320
Vincent Soriano,1 Marion G. Peters,2 and Stefan Zeuzem3
1Department of Infectious Diseases, Hospital Carlos III, Madrid, Spain; 2Division of Gastroenterology, University of California, San Francisco; and 3Medizinische Klinik I, Klinikum der Johann Wolfgang Goethe-Universitat, Frankfurt am Main, Germany
"Antiviral potency, safety issues, posology (i.e., number of pills and how often taken per day), and drug interactions and resistance are the major determinants of success for any of the new specifically targeted antiviral therapies for HCV (STAT-C)......Initial enthusiasm unabated, some concerns regarding the use of STAT-C must be raised. Although there is little doubt about the antiviral potency of most current HCV PIs and polymerase inhibitors, the relatively low genetic barrier for resistance (especially for PIs and nonnucleoside polymerase inhibitors) will require combination with other HCV drugs (for now, this includes pegylated IFN and RBV). This requirement may limit both patient tolerance (because of adverse effects) and efficacy (because of insensitivity toward IFN). However, an advantage is that short courses of pegylated IFN and RBV therapy (12-24 weeks) will make triple-combination treatment more affordable. Emergence of drug resistance in HCV must be considered to be a major threat. Furthermore, many of the resistance mutations to PIs and nucleoside analogues may produce cross-resistance to other compounds within the same drug family, which may complicate rescue-treatment options. The length of persistence of HCV resistance mutations after discontinuation of treatment must be investigated. In addition, most trials have tested new HCV inhibitors on HCV genotype 1; therefore, the efficacy of these drugs against other HCV genotypes needs to be clarified. Finally, the role of new STAT-C molecules in the treatment of nonresponders to IFN-based regimens or patients who experienced relapse has to be defined; although there is a risk of exposure to functional monotherapy and the rapid selection of drug resistance, this subset of patients is the one who most need these drugs."
Chronic hepatitis C virus (HCV) infection remains a global health threat with 175 million carriers worldwide. Currently, treatment consists of pegylated interferon alfa plus ribavirin for 12-72 weeks, depending on HCV genotype, baseline viral load, and initial virological response to therapy. Serious adverse effects and limited sustained virological responses with this therapy warrant the need for novel HCV therapies. Specifically targeted antiviral therapies designed to inhibit the HCV serine protease and the RNA-dependent RNA polymerase have recently entered clinical development. Herein, the main characteristics of these new antiviral agents and the most important challenges arising with their use-namely, toxicities and rapid selection of resistance-are discussed.
Approximately 3% of the worldwide population is infected with the hepatitis C virus (HCV), which represents 175 million people, with 3-4 million individuals newly infected each year [1]. Approximately 70% of infected individuals develop chronic hepatitis C virus infection; among them, 20% will develop cirrhosis within a mean of 20 years. The mortality rate after cirrhosis has developed is 2%-5% per year [1]. End-stage liver disease due to HCV infection currently represents the major indication for liver transplantation in the Western world [2].
Limitations of Current HCV Infection Therapy
A protective vaccine against HCV does not exist, and current, standard therapy consists of the combination of pegylated IFN and ribavirin (RBV), administered for 12-72 weeks [3]. However, only 40%-50% of individuals infected with HCV genotypes 1 or 4 obtain a sustained virological response (SVR), compared with 80% of patients infected with genotypes 2 or 3 who obtain an SVR. Moreover, pegylated IFN and RBV therapy is often associated with important adverse effects, such as depression and anemia [3]. New forms of IFN alfa (i.e., albinterferon) are being tested; these are expected to be more convenient (e.g., administered only twice or even once per month) than the currently used pegylated IFN treatment. However, the results from the first large trials with albinterferon do not appear to improve the SVR rates that are already obtained with pegylated IFN [4]. A prodrug of RBV, taribavirin, was recently tested in randomized clinical trials, and although significantly less anemia was observed with taribavirin than that with RBV, the overall efficacy was unexpectedly lower [5]. A new trial that used higher doses of taribavirin was conducted; efficacy was not significantly increased, but the rate of anemia was diminished [6]. For the growing number of patients for whom current standard therapy for chronic HCV infection has already failed, alternative options, such as maintenance therapy with pegylated IFN [7-9] or retreatment with induction doses and/or extended duration of therapy, have failed to provide significant benefits in most cases.
New HCV Antiviral Agents
The efforts toward developing new compounds against HCV have been hampered by difficulties in replicating the virus in cell culture and the lack of suitable animal models. However, recent advances in the understanding of the HCV genome organization and life cycle and the development of HCV replicons and infectious viral particles in tissue culture systems [10-12] have enabled the rational design of agents that specifically inhibit HCV replication [13-15]. The goal of future HCV treatment is to develop antiviral drugs that are less toxic, more potent, and allow shorter duration of therapy than the current standard of care. Ideally, with these compounds, 12-24 weeks of therapy should be enough to cure most patients who have chronic HCV infection.
Figure 1 schematically represents the life cycle of HCV [16]. Two viral enzymes are currently the most promising targets for the design of specific HCV inhibitors, and several compounds have already been tested against the NS3/4A protease and NS5B polymerase (table 1), some of which will hopefully successfully come to clinical use. Other viral enzymes, such as the helicase, have thus far remained elusive to therapeutic drug discovery and others, such as the NS2 protease and NS5A, have only recently been examined as therapeutic targets.
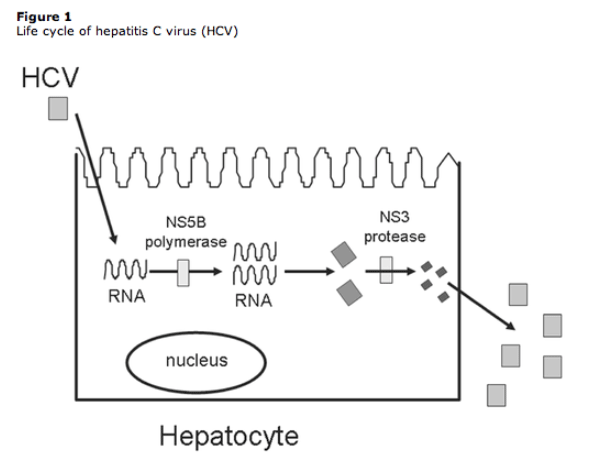
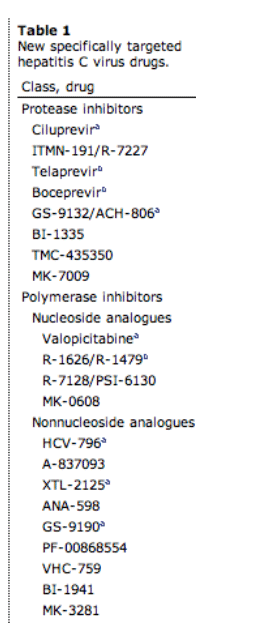
aClinical development has been halted.
bCompound in a more-advanced stage of clinical development.
NS3/4A Serine Protease Inhibitors (PIs)
The amino-terminal domain of the HCV NS3 protein and its cofactor, NS4A, together form a heterodimeric serine protease that cleaves the downstream region of the HCV polyprotein into 4 functional nonstructural proteins, including the HCV polymerase (NS5B) (figure 2). Viral replication can initiate only after all of the individual proteins have been cleaved from the polyprotein. The NS3 protein has also been shown to have nucleotide triphosphatase activity, which is an integral part of the helicase activity; therefore, the NS3 protein acts as a bifunctional enzyme with the activities of both a serine protease and an RNA helicase [17, 18].
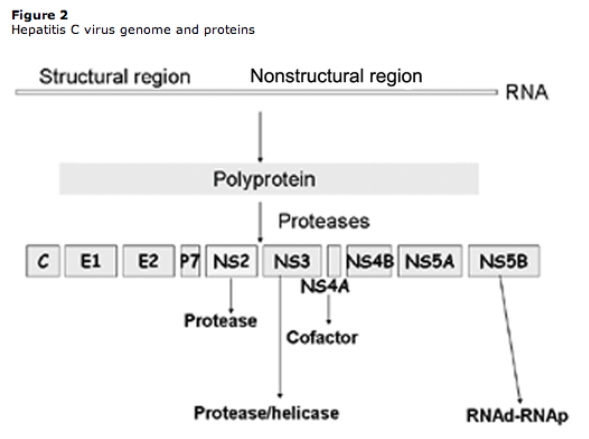
The importance of the NS3/4A serine protease for viral replication has been demonstrated in chimpanzees that were inoculated with genomic HCV RNA containing a mutation in the NS3 protease active site [19]. In addition, a PI that blocks the NS3/4A serine protease prevents viral replication, as proven originally with ciluprevir. This molecule established the first proof of concept when it reduced serum HCV RNA levels by 2-3 log10 IU/mL during 2 days of monotherapy [20, 21]. Additional clinical development was stopped because of cardiotoxicity in animal models [22].
Two major classes of PI molecules against HCV have been developed (table 1). The first group is represented by noncovalent product-based inhibitors, such as ciluprevir and ITMN-191/R-7227. The second group is comprised of covalent reversible inhibitors, also known as serine-trap inhibitors. The most promising drugs in the latest class are telaprevir (TVR) and boceprevir [23].
TVR. TVR is a peptidomimetic NS3/4A serine PI. TVR was discovered using structure-based drug-design techniques [24]. TVR is a covalent but reversible inhibitor of the NS3/4A protease with slow binding and dissociation kinetics [25]. In the subgenomic HCV replicon system, TVR demonstrated a dramatic reduction of viral RNA levels after prolonged exposure, eventually resulting in the elimination of HCV RNA from the replicon cells [26].
In 2006, Vertex started two phase 2 clinical trials, the PROVE-1 and PROVE-2 studies, which recruited patients from the United States and Europe, respectively. The PROVE-1 trial enrolled patients into 4 treatment groups (figure 3). The rate of SVR was 35%-67% in TVR treatment arms, compared with 41% in the control arm. Interestingly, the SVR rate among patients who completed 24 weeks of treatment (61%) was close to that observed among subjects who were treated for 48 weeks (67%). Overall, 12 of 175 patients treated with TVR experienced viral breakthrough with TVR-resistant strains, most of which harbored mutation R155T/I. Interestingly, HCV subtype 1a predominated over subtype 1b in this group of resistant viruses, most likely because 1 nucleotide change is needed to produce the amino acid switch at codon 155 in HCV subtype 1a, whereas 2 nucleotide changes are required to produce the mutation in HCV subtype 1b. Rash, gastrointestinal events, and anemia were the main adverse effects of TVR [27].
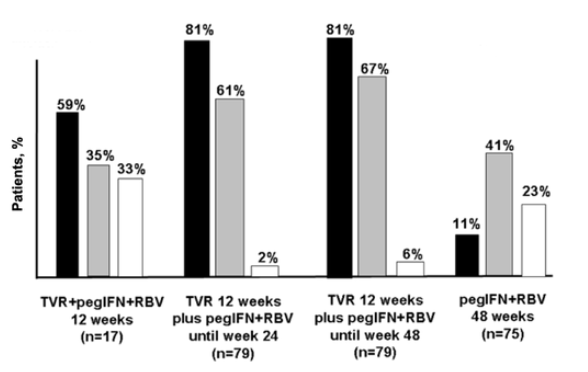
The PROVE-2 trial was conducted in Europe and had a design similar to that of PROVE-1, but with an extra arm that evaluated that combination of pegylated IFN and TVR without RBV. Figure 4 depicts the main results of the trial [28]. In brief, triple therapy (pegylated IFN, TVR, and RBV) for 12 weeks provided significantly more-potent antiviral efficacy at weeks 4 and 12 than did standard pegylated IFN and RBV therapy. A substantial proportion of patients (62%) reached SVR with only 12 weeks of triple therapy. The trial also highlighted the importance of RBV coadministration; only 36% of patients treated with pegylated IFN and TVR for 12 weeks achieved SVR. Moreover, RBV reduced the risk of selecting for TVR-resistant mutations. As in the PROVE-1 trial, skin rashes, nausea, and anemia were the main adverse effects of TVR. It is interesting that the incidence of skin rash associated with TVR increases with time and may affect more than one-third of patients who receive therapy beyond 12 weeks. Viral breakthroughs and selection of resistance were observed in some patients during the first 12 weeks of therapy with TVR, specifically in those patients who were not receiving RBV [29].
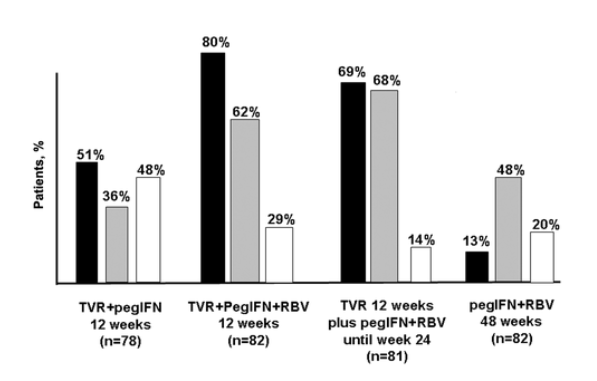
TVR has also been tested in combination with pegylated IFN and RBV in patients who previously were nonresponsive to standard therapy (PROVE-3 trial). Preliminary results have provided encouraging results, which support that in this subset of patients, TVR is not working as only functional monotherapy [30]. TVR is currently being tested in phase 3 clinical trials.
Boceprevir. Boceprevir is another HCV PI that appears to be safe and well tolerated. It binds reversibly to the NS3 protease active site and demonstrates potent activity in the HCV replicon system (effective concentration to achieve 50% of the maximum effect, 0.3-0.4 _mol/L); boceprevir has additive potency when combined with pegylated IFN [31]. In one study, boceprevir (400 mg daily) and pegylated IFN were given to nonresponders infected with HCV genotype 1; the treatment generated an mean decrease in serum HCV RNA levels of >2 log10 IU/mL, with 4 of 10 subjects achieving undetectable HCV RNA levels within 2 weeks [32]. In January 2006, Schering was granted fast-track development status for boceprevir from the US Food and Drug Administration. The initial results in phase 2 trials were somewhat discouraging, most likely because of limitations in study design and treatment dosage. However, in the SPRINT-1 trial, a higher dosage of boceprevir (800 mg 3 times daily) is being tested in combination with pegylated IFN and RBV in 595 patients infected with HCV genotype 1. A subset of patients received a lead-in treatment period with 4 weeks of pegylated IFN and RBV before the addition of boceprevir. Preliminary results are reported in figure 5 [33]. Anemia and gastrointestinal problems are a dose-limiting adverse effect of boceprevir.
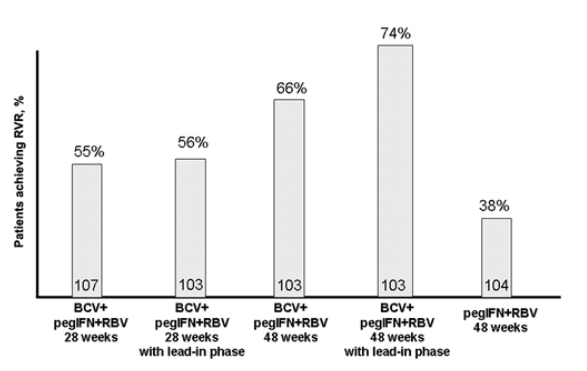
NS5B RNA-Dependent RNA Polymerase Inhibitors
Many antiviral drugs against herpesviruses, hepatitis B virus, or HIV have focused on the viral polymerase, and advances in the treatment of chronic HCV infection are no exception. The HCV RNA-dependent RNA polymerase NS5B is the key viral enzyme responsible for HCV replication (figure 1). It has been extensively characterized at a biochemical and structural level [23, 34, 35]. The NS5B enzyme is thought to form a replicase complex with other viral and cellular proteins in the perinuclear region [34, 36]. Two types of HCV polymerase inhibitors (nucleoside analogues and nonnucleoside analogues) are in development (table 1).
HCV nucleoside analogue polymerase inhibitors-the case of R-1626. R-1626 is a prodrug of R-1479 that was under development by Roche. The majority of R-1626 is converted to R-1479 in the intestinal mucosa [37]. In vitro, R-1479 demonstrated potent antiviral activity against all HCV genotypes, which is consistent with the high sequence conservation at the NS5B nucleotide-binding site [38]. Early in vitro experiments demonstrated moderate synergistic effects when combined with either pegylated IFN or RBV, with no associated increase in cellular toxicity. In a randomized phase 1 study in which 47 patients infected with HCV genotype 1 received R-1626 at a dosage of 3000 or 4500 mg (twice daily for 14 days), the drug produced mean reductions in serum HCV RNA levels of 2.6 and 3.7 log10 IU/mL, respectively [39].
R-1626 gained fast-track evaluation status from the US Food and Drug Administration, and results from a phase 2a trial were recently released (figure 6) [40]. In brief, 2 doses of R-1626 (1500 or 3000 mg twice daily) were given together with pegylated IFN or pegylated IFN plus RBV to 104 IFN-naive patients who were infected with HCV genotype 1. No resistance mutations were observed in patients who had viremia while receiving therapy or after discontination of treatment. However, the incidence of grade 4 neutropenia (<500 neutrophils/μL) was 78% among patients receiving 3000 mg twice daily and 45% among patients receiving 1500 mg twice daily. In addition, 15% of patients developed a mild rash. Moreover, a high rate of HCV infection relapse on completion of therapy was recognized [41]. On the basis of these adverse effects and the drug's limited sustained efficacy, development of R-1626 was halted at the end of 2008.
HCV nonnucleoside analogue polymerase inhibitors. Whereas HCV nucleoside analogues block HCV replication by acting as chain terminators and thus stopping further elongation of the nascent RNA strand, nonnucleoside inhibitors interact with the polymerase outside the catalytic site and produce allosteric changes that critically compromise its function. The HCV polymerase structure shares the same general right-handed configuration of HIV reverse transcriptase, which consists of finger, thumb, and palm domains. Although the polymerase active site is located in the palm domain, to date, at least 5 different sites (named A, B, C, D, and E) have been shown to be targeted by nonnucleoside inhibitors. It is remarkable and unprecedented that >1 of these pockets have been found in the HCV polymerase, but only 1 such pocket has been identified for HIV reverse transcriptase. Mutations that confer resistance to individual drug's binding different sites do not cause cross-resistance to other drugs. In contrast with HIV and hepatitis B virus polymerases, which are RNA-dependent DNA polymerases (or reverse transcriptases), the HCV polymerase works only with RNA as both template and product, and there is no chance for cross-inhibition with HIV and/or hepatitis B virus polymerase inhibitors.
Drug Resistance to HCV Inhibitors
Antiviral potency, safety issues, posology (i.e., number of pills and how often taken per day), and drug interactions and resistance are the major determinants of success for any of the new specifically targeted antiviral therapies for HCV (STAT-C). Of these determinants, drug resistance has emerged as a major threat associated with almost all STAT-C compounds. Because of the error-prone nature of the HCV RNA-dependent RNA polymerase, drug resistance inevitably occurs in patients who are treated with antiviral therapy that is directed to HCV-specific enzymes, which limits its efficacy. Various in vitro studies that have used the HCV subgenomic replicon system have characterized resistance mutations that develop against HCV PIs and polymerase inhibitors. Mutations have most often been identified with use of in vitro selection and less frequently in patients who experienced failed drug therapy in trials. Discordance between the patterns of resistance mutations that are detected in vitro and in vivo is largely dependent on the loss of susceptibility and the fitness of the virus strains that harbor the mutations [42].
Resistance to HCV PIs. Initial studies with ciluprevir demonstrated that molecular clones containing R155Q, A156T, or D168V resulted in 24-, 357-, and 144-fold reductions in drug-susceptibility, respectively, compared with the wild-type replicon [43]. The dominant mutation that is selected with TVR is A156S [44-46], which is at the same position as the mutation that is selected most frequently with boceprevir, A156T [47]. Cross-resistance to TVR and boceprevir has been confirmed with both A156S and A156T, as well as with V36A/M, T54A, and R155Q/T, which may also reduce the susceptibility to these compounds.
Weak cross-resistance to ITMN-191 is demonstrated by the A156T mutation, but ITMN-191 selected for the D168A mutation in vitro [48]. ACH-806/GS-9132 selects for distinct HCV variants that have mutations located at the far amino-terminus of NS3, with no cross-resistance to TVR or boceprevir [23, 49, 50]. Figure 7 reports the most frequent resistance mutations that are detected at treatment failure with the most promising HCV PIs.
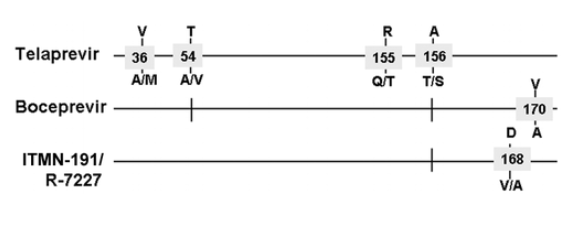
Resistance to HCV polymerase inhibitors. The nucleoside analogue inhibitors appear to select drug resistance mutations through 2 different pathways, both of which are exclusive and modify the active site binding properties. Although 2'-C-methyl-nucleosides generally select for S282T [51-53], 4'-C-methyl-nucleosides select for S96T. In this way, mutations cause cross-resistance to valopicitabine, R-7128, and MK-0608, whereas R-1479 remains active in the presence of S282T, but a 4-fold reduction in susceptibility to R-1479 is observed for S96T mutants [54]. Figure 8 reports the most frequently detected resistance mutations to HCV polymerase inhibitors.
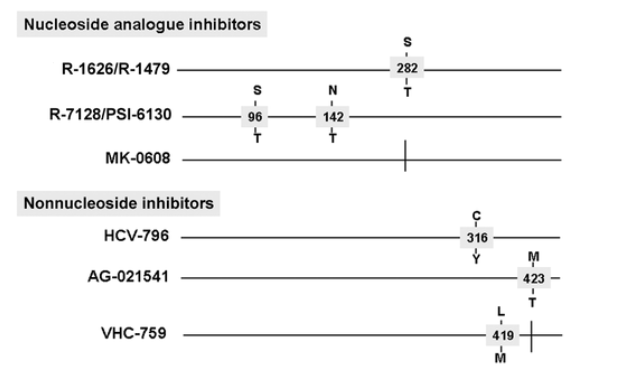
Along with the active site, the HCV polymerase has at least 5 distinct binding sites for compounds that might block its activity. These pockets are located at the thumb (A and B), palm (C and D), and fingers (E) of the right hand-modeled HCV polymerase enzyme (figure 9). Nonnucleoside inhibitors are allosteric blockers and, accordingly, can be grouped into 5 families that display different resistance changes [55-58].
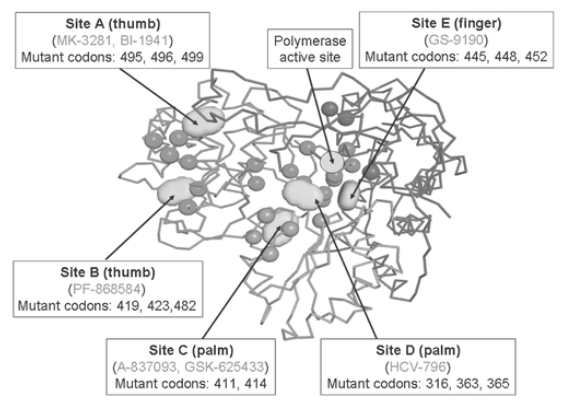
Combination Therapy for Chronic HCV Infection
HCV PIs and nonnucleoside polymerase inhibitors uniformly demonstrate the rapid selection of resistance mutations in most patients when given as monotherapy. In contrast, resistance mutations to nucleoside inhibitors have been selected in vitro, but as yet, this has not been demonstrated in vivo. Thus, nucleoside inhibitors seem to exert a higher genetic barrier for resistance. In any case, drug combinations will be required for treatment of HCV, as has been shown for HIV. It is striking, however, that drug resistance mutations emerge even more rapidly in HCV than they do in HIV, most likely because of the biological characteristics of the HCV life cycle. Viral turnover of HCV involves rapidly vanishing RNA molecules in the cytosol of infected cells, and HCV does not use a life-long genetic reservoir, such as the proviral DNA of HIV or the covalently closed circular DNA of hepatitis B virus. In these other viruses, the viral life span depends on the infected cell survival time, because the genetic material is either integrated into the chromosomes (as for HIV) or maintained as episomes within the hepatocyte nucleus (as for hepatitis B virus). The direct consequence of these biological features is that any drug pressure rapidly drives the selection of preexisting resistant variants of HCV but does so less rapidly for HIV and even more slowly for hepatitis B virus [59].
Another striking observation from phase 2 trials of STAT-C molecules is the recognition of an additive role for RBV, in which it acts as a true antiviral agent [60-62]. The best demonstration of this beneficial interaction comes from the PROVE-2 trial [28]. In that study, the arm that received a triple drug combination (pegylated IFN, RBV, and TVR) significantly outperformed the arm that received just pegylated IFN and TVR, with SVR rates of 69% and 29%, respectively (figure 4). Clearly, RBV should no longer be considered to be a modest accompanying agent, and the mechanism of its action should be definitively elucidated.
Because it is possible to eradicate HCV from the host, it was initially thought that with regard to treatment, "the first will be the winner," which meant that the first HCV drug to gain approval would decrease the chances of success for any other drugs that arrived later. However, as more evidence demonstrates the challenge of drug resistance and the need for drug combinations, it is clear that there will be a place for many treatment compounds. In fact, multiple drugs from distinct drug families will be needed, especially if therapy without pegylated IFN is desired. These drug combinations would ideally be constructed with compounds that exhibit complementary features, such as compounds that have distinct drug resistance profiles targeting either the same enzyme (convergent therapy) or different enzymes (divergent therapy). Table 2 summarizes some of the main features of current STAT-C molecules.
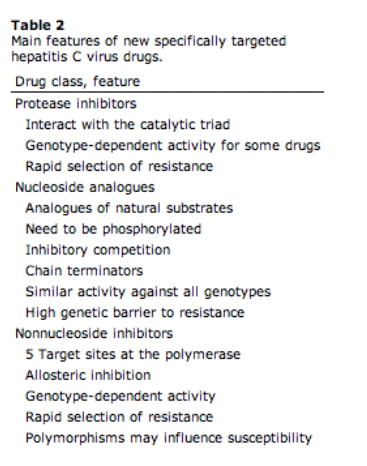
Future Directions
Initial enthusiasm unabated, some concerns regarding the use of STAT-C must be raised. Although there is little doubt about the antiviral potency of most current HCV PIs and polymerase inhibitors, the relatively low genetic barrier for resistance (especially for PIs and nonnucleoside polymerase inhibitors) will require combination with other HCV drugs (for now, this includes pegylated IFN and RBV). This requirement may limit both patient tolerance (because of adverse effects) and efficacy (because of insensitivity toward IFN). However, an advantage is that short courses of pegylated IFN and RBV therapy (12-24 weeks) will make triple-combination treatment more affordable. Emergence of drug resistance in HCV must be considered to be a major threat. Furthermore, many of the resistance mutations to PIs and nucleoside analogues may produce cross-resistance to other compounds within the same drug family, which may complicate rescue-treatment options. The length of persistence of HCV resistance mutations after discontinuation of treatment must be investigated. In addition, most trials have tested new HCV inhibitors on HCV genotype 1; therefore, the efficacy of these drugs against other HCV genotypes needs to be clarified. Finally, the role of new STAT-C molecules in the treatment of nonresponders to IFN-based regimens or patients who experienced relapse has to be defined; although there is a risk of exposure to functional monotherapy and the rapid selection of drug resistance, this subset of patients is the one who most need these drugs.
|
|
|
|
|
|
|