|
Can HIV be cured with stem cell therapy? commentary
|
|
|
Steven G Deeks and Joseph M McCune
Nature Biotechnology July 2010
full text of study in mice:
New Gene Therapy Zinc Fingers Research Published: 'doctors may someday control HIV virus using stem cells without using anti-retroviral drugs' - (07/07/10) Nature Biotechnology
Transplantation of human hematopoietic stem cells engineered to lack the viral coreceptor CCR5 confers resistance to HIV infection in mice.
Antiretroviral therapy has transformed the treatment of HIV infection, but, despite its profound successes, it will not halt the relentless advance of the epidemic. Against this sobering reality, several promising, recent developments in the basic-science arena have led HIV researchers to envision new therapeutic approaches that would completely eradicate the virus, effectively 'curing' HIV disease. In an exciting and impressive display of data published in this issue, Holt et al.1 provide a scientific bellwether for the practical implementation of one such strategy. They show that CCR5, a human gene often required for HIV to enter target cells, can be effectively and permanently disrupted in long-lived, multilineage, human hematopoietic stem cells (HSCs). When introduced into mice, these cells generate an apparently intact human immune system that is resistant to subsequent infection with HIV (Fig. 1a). This result raises the intriguing possibility that HIV-infected individuals might be cured with a one-time infusion of autologous, gene-modified HSCs.
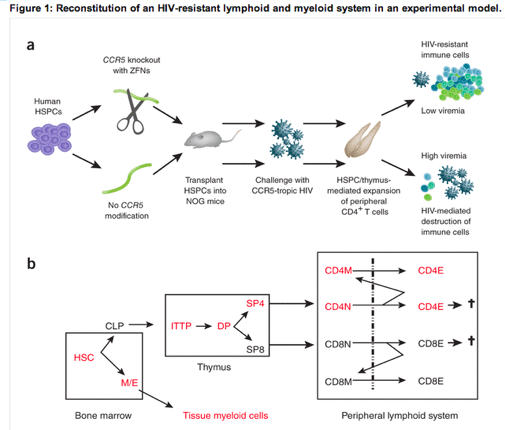
(a) Holt et al.1 isolated human hematopoietic stem/progenitor cells (HSPCs) and used zinc-finger nucleases (ZFNs) to disrupt the CCR5 gene, which is often required for the entry of HIV into target cells. Mice that were successfully engrafted with CCR5-disrupted HSPCs tolerated infection with HIV, whereas those engrafted with unmodified HSPCs exhibited loss of CD4+ T cells and high-level viremia. (b) Long-lived, multilineage hematopoietic stem cells (HSCs) give rise to common lymphocyte progenitors (CLPs) and progenitors of the myelo-erythroid (M/E) lineages. CLPs move through the thymus and differentiate through a series of stages, from CD3-CD4+CD8- intrathymic T progenitor (ITTP) cells to CD3+/-CD4+CD8+ double positive (DP) thymocytes to CD3+ thymocytes that are single positive for CD4 (SP4) or CD8 (SP8) to circulating naïve (N), effector (E), and memory (M) CD4+ or CD8+ T cells. All of the cell stages colored in red can be directly or indirectly disabled by HIV infection.
The introduction of combination antiretroviral regimens against HIV in the mid-1990s was undoubtedly one of the great triumphs of modern medicine. Almost overnight, those who could receive and adhere to the therapies gained a new lease on life. But the passage of time has revealed the limitations of these regimens. Because HIV DNA persists as an integrated genome in long-lived cellular reservoirs, current antiretroviral drugs are unlikely to prove curative2. In addition, the therapies require life-long adherence, which many find challenging, and are often associated with some short-term and long-term toxicity. Moreover, although they suppress HIV replication in a potent, durable manner, they do not restore health; for reasons that remain unknown, treated HIV disease is attended by chronic inflammation, persistent T-cell dysfunction and a shortened life expectancy3, 4. Finally, and perhaps most importantly, antiretroviral therapies and their management are expensive and hard to deliver on a worldwide basis. It is now apparent that the number of HIV-infected people will continue to eclipse the number that can be successfully treated. To stop the epidemic and to provide care for all, a fundamentally different approach is needed.
Gene therapy with HSCs
The concept of HSC-based gene therapy for HIV disease emerged in the epidemic's first decade, when effective antiretroviral regimens were nonexistent. Multiple advances in delivering and expressing transgenes in eukaryotic cells suggested that therapeutic applications were within reach5. Baltimore coined the term "intracellular immunization" to describe the introduction of HIV resistance genes into HSCs to allow long-term repopulation of the host with progeny cells that would be impervious to HIV6. By the late 1980s, startup biotech companies were isolating and preparing human HSCs for transplantation, devising techniques and vectors to genetically modify the cells, and conducting preclinical testing7.
During the same period, studies of HIV pathogenesis were generating data that begged for a therapeutic approach that went beyond antiretroviral drugs. On the one hand, it became clear that CD4+ T-cell depletion, the hallmark of HIV disease, was caused not simply by destruction of late-stage CD4+ T effector cells but also by the host's inability to maintain progenitor cells, including HSCs, intrathymic T progenitor cells and central memory T cells in the periphery8 (Fig. 1b). On the other hand, it was recognized that HIV can persist within multiple lineages of long-lived cells, including T cells and cells of the myeloid lineage (some of which appear to be progenitor cells)2, 9. Taken together, these observations underscored the need to confer HIV resistance to both progenitor cells and their progeny.
Early attempts to engineer HIV resistance into hematopoietic progenitor cells encountered insurmountable hurdles: the scientific and practical constraints of HSC-based therapies were substantial; protocols for genetic modification of HSCs were inefficient and cytotoxic; the preclinical animal models were inadequate; and the choice of anti-HIV genes was driven more by convenience (and/or patent considerations) than by data7. Moreover, it proved difficult to devise a business model that could support the introduction of such a dramatically different, untested and potentially toxic form of therapy into the clinic. More recently, however, two important developments have prompted a reevaluation of HSC-based therapy for HIV: a critical target-the cell-surface receptor CCR5-was identified, and an HIV-infected individual was reported to be virus free in the absence of antiretroviral medications 20 months after receiving a transplant of CCR5-defective allogeneic HSCs10.
Targeting the Achilles' heel of HIV
To enter cells, HIV must bind to either CCR5 or CXCR4, chemokine receptors present on many immune cells11. The vast majority of transmitted viruses use CCR5 (R5 variants). As the disease progresses, HIV evolves and often, but not always, expands its co-receptor preference to include CXCR4 (X4 variants). A small fraction of people carry a 32-base-pair deletion in the CCR5 gene, leading to a truncated gene product, CCR5 Δ32. Those who are heterozygous for CCR5 Δ32 have delayed disease progression after they acquire HIV, whereas homozygotes rarely acquire HIV11. Although lack of CCR5 may be associated with increased risk of developing serious sequela of some uncommon infections12, it does not seem to affect life expectancy and may even be associated with a reduced risk of certain inflammatory diseases. Once the role of CCR5 became clear in the late 1990s, the pharmaceutical industry devoted tremendous resources to the development of small-molecule inhibitors, one of which, maraviroc (Selzentry), is highly effective, well-tolerated and now FDA approved.
This important set of observations inspired several groups to pursue CCR5-targeted gene therapy13, 14. One highly innovative approach relied on engineered zinc-finger nucleases specific for the CCR5 gene15. Such 'molecular scissors' can be delivered to cells ex vivo using methods such as integrase-defective lentiviral vectors, adenoviral vectors and plasmid DNA nucleofection. After specific binding of a pair of zinc-finger nucleases to the CCR5 gene, a double-stranded DNA break is introduced and then repaired by pathways that include error-prone nonhomologous end-joining. This can create a permanent gene disruption that is passed to daughter cells in the absence of persistent transgene expression. The end result is the functional disruption of the CCR5 gene.
Previous work using this approach demonstrated its feasibility in human peripheral blood CD4+ T cells15, and unpublished data from a phase 1 trial suggest that autologous CD4+ T cells modified in this way can be reinfused safely into HIV-infected individuals (P. Tebas, University of Pennsylvania, personal communication). Although of great interest, this strategy does not disrupt CCR5 in HSCs and thus would not enable the long-term generation of both T and myeloid-lineage cells resistant to HIV infection. Evidence supporting such a leap came from another quarter.
The Berlin patient: an instructive N of 1
For all of those engaged in the care and treatment of patients with HIV disease, the world changed in 2009 with the remarkable story of a stably treated, HIV-infected individual-the 'Berlin patient'-who developed acute myeloid leukemia and was transplanted with HSCs from a human leukocyte antigen-matched, homozygous CCR5 Δ32 donor10. Combination antiretroviral therapy was discontinued the day before the transplant. Twenty months later, HIV could not be detected in any of the patient's tissues examined, even when very sensitive techniques were used. Given disappointing treatment outcomes in the past, the HIV research community is hesitant to use the word 'cure', but this single case could very well be the first example to fit the bill.
It is important to emphasize that this road to a cure was arduous and will not be available to the vast majority of patients. The Berlin patient underwent fully ablative conditioning with a potentially lethal regimen that included fludarabine (Fludara), Ara-C, amsacrine (Amerkin, Amsidyl, Amsidine), cyclosporin, mycophenolate mofetil (CellCept), antithymocyte globulin and 4 Gy of total body irradiation. Graft-versus-host disease developed during the post-transplant period. Owing to recurrent acute myeloid leukemia, a second stem cell transplantation using cells from the same donor was performed one year after the first transplant, which again required exposure to myeloablative therapy, including irradiation. No one believes that this approach will soon be used beyond the highly unusual indications for which allogeneic transplantations are normally performed. However, the example of the Berlin patient does provide a strong rationale for the development of CCR5-targeted stem cell therapy.
This case also provides fascinating insights into HIV pathogenesis, some of which may be relevant to future attempts at HIV eradication. For example, it is not entirely clear why HIV did not rebound after combination antiretroviral therapy was discontinued. According to genotypic assays, the patient likely harbored a minority (2.9%) of X4 variant viruses. Also, host-derived CCR5-expressing myeloid cells, which are permissive for HIV infection, persisted for at least five months after the transplant. Given this volatile combination of residual CXCR4-tropic virus and long-lived CCR5-expressing targets, HIV replication and spread should have continued even as the rest of the hematopoietic system was being replaced by homozygous CCR5 Δ32 donor cells.
There are at least two possible explanations for this surprising result. First, the low-level X4 variant may have been a poorly fit dual-tropic virus that was dependent on CCR5 for replication, whereas the number of residual CCR5-expressing myeloid cells was too low to support systemic replication of the CCR5-tropic variants. Second, it is possible that the myeloablative preparative regimen itself contributed to the cure by destroying latently infected T and myeloid cells and by reducing the numbers of susceptible activated CD4+ T cells (HIV more readily infects activated rather than resting target cells). It is also possible that the ongoing graft-versus-host disease may have acted to clear residual susceptible target cells. Detailed exploration of these and other mechanisms will surely provide profound insights into almost any possible intervention aimed at HIV eradication in the future, and should be pursued.
Disruption of CCR5 in autologous HSCs
The strategy of Holt et al.1 is related to the treatment received by the Berlin patient but is potentially relevant to a larger number of patients (Fig. 1a). The authors obtained human CD34+ hematopoietic stem/progenitor cells (HSPCs) (a population enriched in HSCs) from umbilical cord blood and stimulated them to divide with Flt-3 and thrombopoietin. The cells were nucleofected with plasmids expressing CCR5-specific zinc-finger nucleases. A mean of 17% of the cells were successfully modified, 5-7% of which were estimated to be homozygous CCR5-. Modified or unmodified CD34+ cells were then transplanted into nonobese diabetic/severe combined immunodeficient/interleukin 2rγnull (NOD/SCID/IL2rγnull or NOG) mice, a model known to support multilineage human hematopoiesis. As expected, mice engrafted with unmodified stem cells and subsequently challenged with CCR5-tropic HIV (Bal) showed high levels of viremia and loss of peripheral and tissue-based human T cells. Remarkably, in animals repopulated with CCR5-disrupted HSPCs, the virus levels were lower and CD4+ T cells were not depleted, either in the peripheral blood or in the hematolymphoid tissues (e.g., bone marrow, thymus, spleen and small intestine). The preservation of human CD4+ T cells in the experimental group was due to selection for multiple independent clones of successfully gene-modified cells. The frequency of cells containing evidence of CCR5 disruption increased to >80% in the peripheral blood and to >40% in multiple tissues by week 12 of infection.
These experiments raise a number of technical issues and derivative questions. For instance, do genetically modified HSCs confer benefit to a mouse that is already infected (the situation most closely approximating the therapeutic need in humans)? Does a CCR5-disrupted hematopoietic compartment confer protection against infection by X4 viral variants? Is the CCR5-disrupted immune system normal? Are there long-term toxicities that will become evident later? Is off-target cleavage by the zinc-finger nucleases a significant concern (e.g., the CCR2 gene may also be targeted by this nuclease15)? These and other issues can be resolved with further work. In the meantime, the data of Holt et al.1 show convincingly that a relatively small number of gene-modified HSCs can be rapidly selected to ultimately confer resistance to HIV in vivo.
Next steps
If stem cell-based gene therapy for HIV is to become a reality in the clinic, a number of nontrivial theoretical and practical concerns must be addressed. First, in the current era, when clinicians are increasingly concerned about the 'toxicity' of ongoing viral replication, will patients and their healthcare providers be willing to allow HIV to replicate at high levels in the absence of antiretroviral therapy so that CCR5-deficient cells can be selected? There is now a growing consensus that HIV replication causes significant and perhaps irreversible harm to many organs, including those of the cardiovascular, renal, hepatic and neurologic systems4, so this approach must be assumed to carry some risk.
Second, will a partially effective antiviral intervention (which is what the gene-modified cells represent) select for the outgrowth of a resistant virus population, such as X4 variants? The history of HIV therapeutics is absolutely clear on this issue: if HIV is allowed to replicate in the presence of a selective pressure, it will find a way to survive. This concern is even more pressing as it is widely believed that X4 variants are more virulent than R5 variants. Although X4 variants are only infrequently selected in patients treated with small-molecule CCR5 inhibitors (e.g., maraviroc), this is only true when a fully suppressive regimen is used from day one. It is not likely that transplantation of gene-modified HSCs will be fully suppressive at first, particularly if partially myeloablative therapy is used.
Third, will ablative therapy be needed to allow stem cell engraftment and, if so, will short- and long-term toxicity preclude its use in those most likely to be offered this intervention first? Those most in need of aggressive interventions typically have dual-tropic virus and are therefore unlikely to respond to any approach based on disruption of CCR5 (ref. 16). And with advanced disease, they have a paucity of HSCs and damaged hematopoietic microenvironments (such as bone marrow, thymus and lymph node) that would normally support the maturation of modified HSCs.
Finally, the mechanism whereby HIV causes CD4+ T-cell depletion remains unclear8. Although HIV can clearly kill cells directly, many if not most cells in an HIV-infected individual die as a consequence of indirect viral effects. Generalized activation of the immune system, for example, is harmful to the function of T and myeloid cells and to the regeneration of multiple lineages. These indirect effects may persist even as the virus is driven to extinction by the gradual emergence of an HIV-resistant T-cell population.
Reaching for blue sky
Although the above concerns are daunting, the epidemic is not going to disappear, the science of stem cells is becoming more tractable, sociopolitical forces are forging new perspectives in healthcare, and now is not the time to stop. From our perspective, HSC-based gene therapy for HIV disease may make a significant impact on the worldwide epidemic if two goals can be met. First, it is essential to find a way to deliver these therapies to all in need, in a manner that is safe, affordable and generally available around the world. Many clever approaches to do this have been proposed in the past, and more will surely emerge. Second, the preclinical and clinical development of these strategies requires a sustainable financial model. Such a model may involve reprioritization of governmental efforts, creative plans to incentivize existing pharmaceutical and healthcare delivery systems, and global assistance programs motivated by a common desire for a world free of HIV. This may seem like a formidable exercise, but it is worth noting that if one-shot, modified HSC-based gene therapy can be made efficacious and accessible in the context of HIV disease, similar approaches will likely be applicable to a host of other chronic diseases, infectious and otherwise. If so, the treatment paradigms of the future will look vastly different from today's. In the same way that problems associated with the reliance on fossil fuels have stimulated the development of alternative strategies of energy delivery, so too may the ongoing crisis in the HIV epidemic spark novel approaches to the provision of healthcare in the future.
Conclusion
The progress in HIV therapeutics over the past 15 years has been tremendous. The life expectancy of most people who present with HIV disease today in resource-rich regions is on the order of decades. Yet antiretroviral drugs have intrinsic limitations that are unlikely to be surmounted. What is needed, therefore, is a 'game changer', such as a cure for HIV infection or an effective vaccine. Could a one-shot manipulation of HSCs be the answer? We will not know unless we continue to move these new technologies into the clinic. Even if CCR5-targeted gene therapy is not the ultimate solution, human studies are certain to be highly informative with regard to HIV pathogenesis and human immunology.
Affiliations
Steven G. Deeks and Joseph M. McCune are in the Department of Medicine, University of California, San Francisco, California, USA.
Competing financial interests
S.G.D. has provided advice to Sangamo for the development of a phase 1/2 clinical study aimed at defining the role of CCR5 gene-modified mature T cells in HIV disease, and is currently participating without compensation on a DMSB for an ongoing investigator-initiated study at the University of Pennsylvania in which this technology is being explored.
Corresponding authors
Correspondence to:
* Steven G Deeks (sdeeks@php.ucsf.edu) or
* Joseph M McCune (mike.mccune@ucsf.edu)
References
1. Holt, N. et al. Nat. Biotechnol. 28, 839-847 (2010).
2. Siliciano, J.D. et al. Nat. Med. 9, 727-728 (2003).
3. Kuller, L.H. et al. PLoS Med. 5, e203 (2008).
4. Phillips, A.N., Neaton, J. & Lundgren, J.D. AIDS 22, 2409-2418 (2008).
5. Friedman, A.D., Triezenberg, S.J. & McKnight, S.L. Nature 335, 452-454 (1988).
6. Baltimore, D. Nature 335, 395-396 (1988).
7. Rossi, J.J., June, C.H. & Kohn, D.B. Nat. Biotechnol. 25, 1444-1454 (2007).
8. McCune, J.M. Nature 410, 974-979 (2001).
9. McCune, J.M. Cell 82, 183-188 (1995).
10. Hutter, G. et al. N. Engl. J. Med. 360, 692-698 (2009).
11. Moore, J.P., Kitchen, S.G., Pugach, P. & Zack, J.A. AIDS Res. Hum. Retroviruses 20, 111-126 (2004).
12. Glass, W.G. et al. J. Exp. Med. 203, 35-40 (2006).
13. DiGiusto, D.L. et al. Sci. Transl. Med. 2, 36ra43 (2010).
14. Shimizu, S. et al. Blood 115, 1534-1544 (2010).
15. Perez, E.E. et al. Nat. Biotechnol. 26, 808-816 (2008). w
16. Hunt, P.W. et al. J. Infect. Dis. 194, 926-930 (2006).
| |
|
|
|
|
|