|
Achieving hepatitis C elimination in Europe - To treatment scale-up and beyond
|
|
|
Download the PDF here  
Download the PDF here  
"A second and potentially more complex barrier to treatment scale-up is countries' capacities and health infrastructures to deliver treatment and prevention programs at scale. If Fraser and colleagues' model predictions are correct - that elimination is theoretically achievable by scaling up prevention and treatment -broader questions must now be asked of these settings. How will countries scale up access to treatment, and what additional health system strengthening activities would be required if unrestricted access were granted tomorrow? These activities include training and education for providers, increasing laboratory capacity to ensure appropriate testing and increasing awareness of DAAs among key risk populations. In addition, could these changes to the health system be started now in anticipation of drug prices that will inevitably decrease? Mathematical models suggest that the extent and speed at which treatments are scaled-up are important determinants of reinfection and cumulative treatment costs. Lessons learnt from implementation science must therefore be incorporated into broader hepatitis C strategies. In settings with unrestricted access, such as Australia, evidence is emerging that the additional activities required to achieve and sustain high treatment rates require substantial time and effort to coordinate. This includes addressing staffing capacity constraints, diversifying and decentralising services, addressing stigmatization and discrimination and ensuring geographically and culturally appropriate services are available."  
----------------------------------------  
Model projections on the impact of HCV treatment in the prevention of HCV transmission among people who inject drugs in Europe - see below following editorial  
Editorial - Achieving hepatitis C elimination in Europe - To treatment scale-up and beyond  
Jnl of Hepatology March 2018 - M. Hellard1,2,3,⇑, N. Scott1,2, R. Sacks-Davis1,4, A. Pedrana1,2
1Burnet Institute, Melbourne, VIC 3004, Australia; 2Department of Epidemiology and Preventive Medicine, Monash University, Clayton, VIC
3008, Australia; 3Department of Infectious Diseases, The Alfred Hospital and Monash University, Melbourne, VIC 3004, Australia; 4Department of Medicine, University of Melbourne, Parkville, VIC 3010  
Fraser and colleagues1 have used a mathematical model to show that for many European countries, using direct-acting antiviral (DAA) therapy to treat hepatitis C infection is unlikely to have a substantive impact on hepatitis C incidence and prevalence among people who inject drugs (PWID) unless treatment rates are increased substantively beyond current levels. Their model explicitly accounts for the opioid substitution therapy (OST) and needle and syringe program (NSP) coverage of each setting, which is important not only because of the proven effectiveness of the programs in reducing hepatitis C transmission,2 but because it allows the authors to quantify the benefits of prevention interventions in isolation and when combined with treatment scale-up. Their findings reiterate the importance of high prevention coverage (OST and NSP) as a primary method of reducing incidence and prevalence and also as a method of enhancing the epidemiological impact of treatment, through treatment-as-prevention.  
The implication of this work is that for many countries the elimination of hepatitis C as a public health threat is not going to happen by chance. A number of barriers need to be overcome, requiring a focused effort from governments and health services. The first barrier is that widespread treatment scale-up is currently precluded by restricted and inconsistent access policies in many European countries.[3], [4] Fraser and colleagues' model predicts that without scaling up universal treatment access, OST and NSP alone can produce notable decreases in hepatitis C prevalence, from approximately 20% to more than 75%, depending on current coverage. However, the greatest and most rapid benefits require a combination of both prevention and treatment scale-up in unison.  
Despite the current barriers to treatment access in some countries, continued falling prices and growing evidence for the cost-benefit of elimination efforts means that treatment access is constantly changing. It is difficult to imagine that universal treatment access will not become a reality for these countries eventually. Therefore, while the continued advocacy for unrestricted treatment access continues to be of immediate importance, it should not be allowed to distract from the many other aspects of a comprehensive programmatic response that are required to achieve hepatitis C elimination - activities which represent equally critical pieces of the elimination puzzle.  
A second and potentially more complex barrier to treatment scale-up is countries' capacities and health infrastructures to deliver treatment and prevention programs at scale. If Fraser and colleagues' model predictions are correct - that elimination is theoretically achievable by scaling up prevention and treatment -broader questions must now be asked of these settings. How will countries scale up access to treatment, and what additional health system strengthening activities would be required if unrestricted access were granted tomorrow? These activities include training and education for providers, increasing laboratory capacity to ensure appropriate testing and increasing awareness of DAAs among key risk populations. In addition, could these changes to the health system be started now in anticipation of drug prices that will inevitably decrease? Mathematical models suggest that the extent and speed at which treatments are scaled-up are important determinants of reinfection and cumulative treatment costs. Lessons learnt from implementation science must therefore be incorporated into broader hepatitis C strategies. In settings with unrestricted access, such as Australia, evidence is emerging that the additional activities required to achieve and sustain high treatment rates require substantial time and effort to coordinate. This includes addressing staffing capacity constraints, diversifying and decentralising services, addressing stigmatization and discrimination and ensuring geographically and culturally appropriate services are available.[5], [6]  
Health system limitations form an upper bound on the possible treatment scale-up, since existing services may not have the resources or workforce capacities to support substantive increases in patient numbers. A third barrier to scale-up is effectively engaging key risk populations and their specific needs.7 Implicit in Fraser and colleagues' study is the well-established message that to achieve hepatitis C elimination in most European countries treatment scale-up needs to happen among PWID, the group at greatest risk of transmission, otherwise treatment-as-prevention will not be effective.  
However, decades of conservative drug policy in most European countries, leading to stigmatization and discrimination, among other factors, have resulted in low engagement of PWID in care in many communities.[8], [9], [10] This must be addressed, since improvements in all aspects of the care cascade,11 including increased testing frequency well beyond current practice,12 are required to achieve and sustain high treatment uptake among PWID.
The establishment of flexible and culturally acceptable models of care for patients, in particular PWID, has been shown to improve both capacity and engagement. The simplicity of treatments means they can be administered in primary healthcare settings, rather than confined, as in the era of pegylated interferon and ribavirin, to hospital settings. There is increasing evidence that community-based treatment approaches are highly effective in engaging PWID in care and treatment.[13], [14] In Australia, a policy enabling non-specialist prescribing of hepatitis C treatments has been in place since DAAs became available in 2016. As a result, over the first 14 months 46% of treatments were prescribed by non-specialists,13 with particularly high rates of non-specialist prescribing among indigenous, immigrant and regional communities. This policy also facilitates the use of peer-led15 and nurse-led models of care,16 which are able to use community-based services[17], [18] as primary contact points for diagnosis and treatment.  
Fraser and colleagues also touch on the importance of robust surveillance systems for monitoring and evaluating any hepatitis response. In addition to the capacity to monitor chronic HCV prevalence and treatment rates among PWID, these systems will need to monitor newly acquired infection, including reinfection after treatment.19 These systems are also critical to ensure services are appropriately located. For example, transmission occurring through injecting drug use is geographically heterogeneous and related to drug market characteristics, yet while health services are also often geographically heterogeneous, they are often distributed according to different coverage objectives. Therefore, it is important that effective resource planning is undertaken to ensure appropriate hepatitis C-related services are located in areas of greatest demand and where unmet need is greatest. This should be guided by evidence and appropriate data, and again, it requires time and planning to put suitable systems in place.20  
In summary, Fraser et al. demonstrate that substantive gains can be made towards hepatitis C elimination in Europe if increases in treatment rates and harm reduction coverage are achieved. Universal access to DAAs is required to achieve the WHO 2030 elimination targets and ultimately this will happen in the region. However, while waiting for this to occur, countries should act now to improve their health services infrastructure and ensure the platforms required are in place to successfully deliver scaled-up DAA treatment in a timely manner when universal access does arrive. A comprehensive elimination program requires more than just DAAs, and depending on the starting point it can take many years to set up sufficient service diversity, infrastructure capacity and surveillance systems to facilitate the increases in testing and retention in care that are required. Lessons should be learnt from other settings on the most effective models of care to maximize treatment uptake.  
---------------------------  
"Main findings  
Treatment scale-up is needed to achieve observable reductions in chronic HCV prevalence among PWID in most sites in Europe, even with new DAAs. Doubling the DAA treatment rates may lead to observable reductions (12-24% decrease) in chronic prevalence by 2026 in Belgium, Denmark, Hamburg, Norway, and Scotland; but not in Finland or Sweden. Exceptions include Czech Republic, Slovenia, and Amsterdam, which, at current HCV treatment rates, are projected to reduce chronic HCV prevalence from a third to a half by 2026. This is because of the low or decreasing prevalence of infection in these settings. Alternatively, increasing OST and NSP coverage to 80% with the current HCV treatment rates would reduce chronic HCV prevalence by 17-20% in Finland and Hamburg, and 30-79% in all other sites. Reducing HCV incidence to <2% by 2026 requires little action in Amsterdam, Czech Republic, and Slovenia, whereas in Belgium, Denmark, Hamburg, Norway, and Scotland it will require at least a fivefold increase in the current HCV treatment rates, or 1.8- to 4.7-fold if OST and NSP are scaled-up to 80% coverage."  
"Lay summary  
Measuring the amount of HCV in the population of PWID is uncertain. To reduce HCV infection to minimal levels in Europe will require scale-up of both HCV treatment and other intervention that reduce injecting risk (especially OST and provision of sterile injecting equipment)."  
Model projections on the impact of HCV treatment in the prevention of HCV transmission among people who inject drugs in Europe  
Jnl of Hepatology March 2018 - Hannah Fraser, Natasha K.Martin, Henrikki Brummer-Korvenkontio, ..., Ruth Zimmermann, Peter Vickerman, Matthew Hickman  
Highlights  
o Chronic HCV prevalence and treatment rates among PWID vary widely across Europe.
o HCV treatment scale-up is required in most sites to reduce HCV transmission.
o Increasing OST/NSP coverage enhances HCV treatment prevention benefit.  
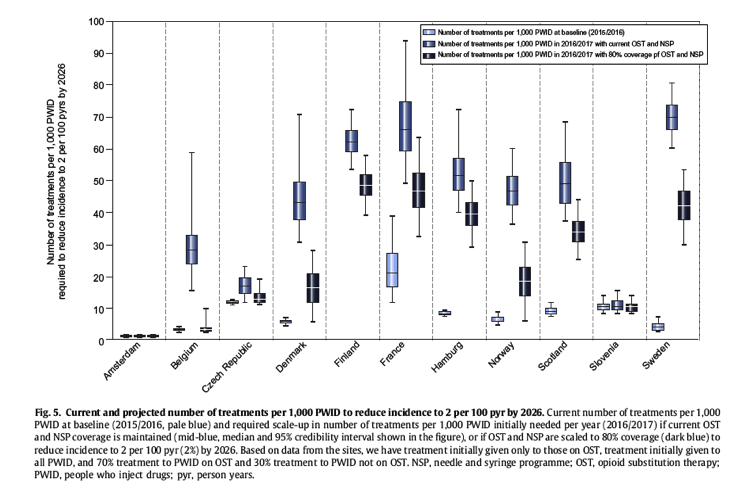
Background & Aims  
Prevention of hepatitis C virus (HCV) transmission among people who inject drugs (PWID) is critical for eliminating HCV in Europe. We estimated the impact of current and scaled-up HCV treatment with and without scaling up opioid substitution therapy (OST) and needle and syringe programmes (NSPs) across Europe over the next 10 years.  
Methods  
We collected data on PWID HCV treatment rates, PWID prevalence, HCV prevalence, OST, and NSP coverage from 11 European settings. We parameterised an HCV transmission model to setting-specific data that project chronic HCV prevalence and incidence among PWID.  
Results  
At baseline, chronic HCV prevalence varied from <25% (Slovenia/Czech Republic) to >55% (Finland/Sweden), and <2% (Amsterdam/Hamburg/Norway/Denmark/Sweden) to 5% (Slovenia/Czech Republic) of chronically infected PWID were treated annually. The current treatment rates using new direct-acting antivirals (DAAs) may achieve observable reductions in chronic prevalence (38-63%) in 10 years in Czech Republic, Slovenia, and Amsterdam. Doubling the HCV treatment rates will reduce prevalence in other sites (12-24%;  
Belgium/Denmark/Hamburg/Norway/Scotland), but is unlikely to reduce prevalence in Sweden and Finland. Scaling-up OST and NSP to 80% coverage with current treatment rates using DAAs could achieve observable reductions in HCV prevalence (18-79%) in all sites. Using DAAs, Slovenia and Amsterdam are projected to reduce incidence to 2 per 100 person years or less in 10 years. Moderate to substantial increases in the current treatment rates are required to achieve the same impact elsewhere, from 1.4 to 3 times (Czech Republic and France), 5-17 times (France, Scotland, Hamburg, Norway, Denmark, Belgium, and Sweden), to 200 times (Finland). Scaling-up OST and NSP coverage to 80% in all sites reduces treatment scale-up needed by 20-80%.  
Conclusions  
The scale-up of HCV treatment and other interventions is needed in most settings to minimise HCV transmission among PWID in Europe.  
Lay summary  
Measuring the amount of HCV in the population of PWID is uncertain. To reduce HCV infection to minimal levels in Europe will require scale-up of both HCV treatment and other interventions that reduce injecting risk (especially OST and provision of sterile injecting equipment).  
Introduction  
Chronic hepatitis C virus (HCV) infection is a leading cause of liver disease and morbidity, causing more deaths than HIV in the United States and other high-income countries.[1], [2], [3], [4] Preventing HCV transmission among people who inject drugs (PWID) is critical for averting future liver disease in Europe and elsewhere5 and new HCV infections in this group.6 Primary prevention through opioid substitution therapy (OST) and high-coverage needle and syringe programmes (NSPs) can reduce HCV transmission among PWID[7], [8] and averts new HCV infections,9 but substantial reductions in HCV prevalence are unlikely to be achieved without scaling-up HCV treatment.[10], [11], [12], [13], [14], [15]  
The arrival of highly effective and short-duration direct-acting antivirals (DAAs) with cure rates (sustained viral response [SVR]) above 90% for all genotypes has made HCV "treatment as prevention" more than a theoretical possibility.[16], [17], [12] However, the current high cost of DAA regimes (often >€30,000 per treatment regime in higher-income countries) is a barrier to scaling-up treatment in most countries. European guidelines previously recommended prioritising DAAs for patients with advanced liver disease. Now, they suggest that HCV treatment should also be provided to people with a risk of transmitting HCV, such as PWID.[18], [19] A recent economic model suggested that, in general, it is more cost effective to delay treatment of a mild disease until more moderate stages of fibrosis.20 However, when these individuals have an ongoing transmission risk, they should be prioritised for early treatment over other patient groups.21  
In this paper, we estimate the current HCV treatment rates and coverage of OST and NSP in PWID across 11 sites in Europe. We assess the impact of these and scaled-up HCV treatment rates and other primary prevention on HCV prevalence and incidence over the next 10 years.  
Materials and methods  
Model  
We used a dynamic deterministic mathematical model of HCV transmission among PWID, stratifying PWID according to intervention status (no OST or NSP, on OST, NSP, or both) alongside HCV infection and treatment status (susceptible and never infected, previously infected, chronically infected, on treatment, and treatment failure[9], [10]). In three sites (Czech Republic, Finland, and Sweden), PWID are also stratified by drug type (opioid or methamphetamine/amphetamine). PWID enter the model through a constant rate that individuals initiate injecting. All PWID are assumed initially susceptible to HCV infection (Fig. 1A). Susceptible PWID can become infected at a per capita rate proportional to the background prevalence of the disease, which changes as HCV treatment increases. Transmission is reduced by a fixed multiplicative cofactor dependent on OST and NSP status (Fig. 1B). Once infected, PWID either transition to the chronically infected group (Ab+, RNA+), or spontaneously clear infection and transition to the previously infected group (Ab+, RNA−). This previously infected group is assumed to be reinfected and clear infection at the same rate as susceptible PWID.[11], [22], [23] Chronically infected PWID (both primary and reinfection) can be treated. If treatment is successful and SVR is attained, PWID transition to the previously infected group. However, if SVR is not attained, PWID transition to the treatment-failure group. In the baseline model, treatment failures cannot be retreated (Fig. 1A). Once treatment is switched to DAAs, we assume that treatment failures can be retreated. PWID leave the model through permanent cessation of injecting, or drug-related or non-drug-related mortality. All PWID enter the model with no coverage of OST or NSP, and transition between the different intervention states (OST and/or NSP) at site-specific fixed per capita rates (Fig. 1B; Tables S1a-S1k). Further details of the model, including model equations, are in the Supplementary materials.  
Model parameterisation and calibration  
The model was parameterised to each of the 11 sites (see Table 1 and Tables S1a-S1l for site-specific information).  
For sites with opioid injecting only, 2,500 model parameter sets were randomly sampled from the parameter uncertainty distributions (see Tables S1a-S1l). For each parameter set, the model was fit to the PWID population size by varying the rate that individuals initiate injecting, to OST and NSP coverage levels by varying the recruitment rates onto OST and NSP, and to either the chronic or antibody HCV prevalence at a site-specific time point by varying the transmission rate. For Czech Republic, Finland, and Sweden, the model was fit to more parameters (see Supplementary materials for further details). HCV incidence was estimated from model inputs assuming a stable epidemic, except for Amsterdam where additional data were available, suggesting a decreasing PWID population size and declining incidence.15  
In sites with opioid and methamphetamine/amphetamine injecting, we assume that the baseline risk of HCV transmission is the same for all PWID.[24], [25], [26] There is an equal NSP coverage across both types of injectors, but only opioid users can be recruited into OST.  
In all but four sites (Czech Republic, Finland, Sweden, and Norway), HCV treatment of PWID was modelled only among those on OST for initial analyses, as in these sites only PWID on OST are currently treated.  
Model projections and analyses  
Data on PWID treatment numbers for each site were scaled to give a rate per 1,000 PWID, as well as the percentage of chronic HCV infections treated in 2015/2016. By scaling to give a rate based on the total PWID population size, we can easily compare the current and projected future treatment numbers between all sites. All known increases in treatment prior to 2015 were included in the model.  
We used the model to project the change in prevalence and incidence between 2016 and 2026 if treatment is switched from interferon-based therapies to new DAAs (SVR rate 90% [85-95%]). Current treatment rates per 1,000 PWID either are maintained, doubled, or increased to 50 per 1,000 PWID treated annually. Impact projections either assumed that current coverages of OST and NSP are maintained, or OST and NSP are scaled-up to 80% coverage (if not already achieved). We determined the annual treatment number (expressed as a rate of treatment per 1,000 PWID) needed to reduce incidence to 2 per 100 person years (pyr) by 2026. This is the number of treatments annually per 1,000 PWID and is, therefore, constant when projecting to 2026.  
We estimated the z-score associated with the mean difference in chronic prevalence given the uncertainty in chronic HCV generated by the model. We categorised a z-score <0.5 as a modest change (unlikely to be observed), between 0.5 and 1.5 as a moderate change (may be observable), and scores greater than 1.5 or 3.0 as changes that are increasingly and highly likely to be observed.  
Uncertainty analysis  
To consider the effect of uncertainty within the underlying parameters, we performed a linear-regression analysis of covariance on the relative decrease in HCV prevalence and incidence between 2016 and 2026 when current treatment rates are doubled. For each site, the proportion of the sum of squares contributed by each parameter of each model outcome was calculated to estimate the importance of each parameter to the uncertainty.27
For further details regarding the materials used, please refer to the CTAT table and Supplementary information.  
Results  
Baseline HCV treatment rates  
HCV treatment of PWID started at different times across the sites, ranging from 1997 (Slovenia) to 2009 (Norway) with very few PWID having been treated in Finland. In Fig. 2, the percentage of chronic HCV prevalent cases among PWID that were treated in 2015/2016 based on data from each site is shown. They vary from <0.1% in Finland to 0.5-2% in Sweden, Denmark, Belgium, Norway, and Amsterdam, and to >5% in Czech Republic and Slovenia.  
Model projections  
Chronic HCV prevalence among PWID  
At baseline in 2016, the projected chronic prevalence varied from <25% in Czech Republic (20.9% [95% CI 18.2-23.8%]) and Slovenia (16.2% [10.7-21.7%]) to >55% in Finland (56.1% [53.1-59.4%]) and Sweden (60.0% [57.4-62.9%]). In Fig. 3, the projected baseline and 10-year chronic HCV prevalence among PWID in each setting for different levels of scale-up of HCV treatment with new DAAs are shown. In Fig. 4, the same projections are shown, but with scale-up in OST and NSP coverage to 80%.  
Switching to DAAs with treatment rate maintained  
In the majority (8/11) of the sites, the difference in projected chronic HCV prevalence after 10 years if the current treatment rates with DAAs remain constant is <5%. In these sites, the median absolute difference ranges from <1.5% in Finland, Sweden, and Belgium up to 3-4% in Norway, Denmark, France, Hamburg, and Scotland. This difference is substantially smaller than the uncertainty in the baseline chronic HCV prevalence in the sites. This equates to a relative decrease of <10% at each site (see Supplementary materials).  
In the remaining three sites (Amsterdam, Czech Republic, and Slovenia), there is a much greater relative decrease in chronic HCV prevalence between 2016 and 2026 from switching to DAAs: 37.5% (26.6-51.8%) in Czech Republic and 49.3% (25.0-98.0%) in Slovenia. In Amsterdam, the decreasing population size and concurrent decrease in transmission contribute around 90% of the relative decrease of 51.8% (28.7-65.7%) in chronic prevalence between 2016 and 2026. These sites have a z score >3.0, indicating that an observable change in chronic prevalence will likely occur by switching to DAAs with the current treatment rates.  
If all sites switched to DAAs with the current treatment rates and concurrently increased OST and NSP coverage to 80%, the model projects a reduction in prevalence in all sites. This reduction is from <20% in Finland (17.6% [10.0-27.9%]) and Hamburg (19.5% [11.7-27.6%]), 30-50% in Scotland, Sweden, France, Norway, Denmark, and Belgium, to >50% in Czech Republic and Amsterdam, and >75% in Slovenia. The differential benefit of scaling-up OST and NSP alongside treatment on reducing chronic HCV prevalence ranges from >10 to <1.5 times because of baseline coverage. For example, in Finland, Sweden, and Belgium, scaling-up OST and NSP with the current HCV treatment rates reduces chronic HCV prevalence in 10 years by 17.1%, 31.1%, and 48.4%, respectively, compared to 0.1%, 1.8%, and 4.4% reduction without OST and NSP scale-up. In contrast, there is only a small projected improvement in Amsterdam and Czech Republic, which already have high coverage of OST and NSP (Tables S1a-S1k). Other sites are projected to improve reductions in chronic HCV prevalence from two to three times (Slovenia, Hamburg, and Scotland) and five to six times in France, Denmark, and Norway (Table S3).  
Switching to DAAs with treatment rate doubled  
For sites with high baseline chronic prevalence (>55% at baseline) and low treatment rates (<1% of chronic infections treated at baseline) (e.g. Sweden and Finland), doubling the DAA treatment rates has little effect on the projected prevalence in 2026 (0.4% [0.3-0.6%] and 5.2% [3.3-10.4%] relative decrease, respectively) if OST and NSP are maintained at current coverage. For sites with moderate chronic prevalence (30-50% at baseline) and <2.5% of chronic infections being treated annually in 2015/2016 (Belgium, Denmark, Hamburg, Norway, and Scotland), doubling the DAA treatment rates could reduce chronic HCV prevalence from 11.6% relative decrease (Belgium) up to 23.5% (Scotland).  
France has a moderate chronic prevalence (47.3%) at baseline and high initial treatment rate (4.5% [2.4-8.3%] of all chronic infections treated annually). When their treatment rate is doubled with DAAs, this yields a greater relative decrease in chronic prevalence than other sites with moderate prevalence (36.4% [16.7-85.5%]). The credibility intervals are wide because of uncertainty in the estimates of HCV treatment rates.  
In Czech Republic and Slovenia, doubling the DAA treatment rates is projected to reduce chronic prevalence by >90% (Fig. 3), and in Amsterdam by 55.8% (32.8-69.6%).  
Increasing OST and NSP to 80% coverage and doubling the DAA treatment rates are projected to reduce chronic prevalence between 17.9% (10.3-28.2%) in Finland and 99.5% (91.8-99.9%) in Slovenia. In sites with high baseline treatment rates (Czech Republic and Slovenia), the decrease in prevalence is primarily caused by doubling treatment rates (97.3% and 91.6% decrease in Czech Republic with and without scaled-up OST and NSP, respectively, and 99.5% and 97.4% in Slovenia). For sites with low baseline treatment rates and low coverage of OST and NSP, it is the increase in OST and NSP that drives the decrease in chronic prevalence rather than the doubling in treatment rates. In Finland, the decrease changes from 0.4% to 17.9% when additionally scaling-up OST and NSP, from 5.2 to 35.5% in Sweden, and from 11.6% to 55.6% in Belgium.  
DAA treatment rate 50 per 1,000 PWID  
Increasing the annual DAA treatment rates to 50 per 1,000 PWID with the current OST and NSP coverage leads to substantial reductions in chronic HCV in all sites (Fig. 3). In the high-prevalence sites of Finland and Sweden, chronic prevalence reduces by about half by 2026. Conversely, chronic HCV prevalence decreases by 70% or more in most moderate-prevalence sites (Belgium, Hamburg, Scotland, Norway, and Denmark). In France, however, the decrease is smaller and more uncertain (47.6% [21.7-73.8%]). In low-prevalence settings (Czech Republic and Slovenia), chronic prevalence is projected to decrease by around 99% by 2026.  
In projections with OST/NSP scale-up to 80%, prevalence decreases by more than three quarters in all sites, with 7/11 sites (Scotland, Denmark, Norway, Belgium, Amsterdam, Czech Republic, and Slovenia) projecting a decrease of >95%.  
HCV incidence among PWID  
Baseline projections of incidence before 2015 agree with observed incidence estimates where data were available (Supplementary materials). Projected changes in incidence from 2016 to 2026 are shown in Figs. S2 and S3, without and with scale-up of OST and NSP to 80% coverage using DAAs. HCV incidence is projected to remain largely unchanged with the current OST and NSP coverage in all but three sites if the current HCV treatment rates are maintained using DAAs. However, if OST and NSP are scaled-up to 80% coverage, projections estimate a relative decrease in incidence of over 35% at all sites.  
The treatment number per 1,000 PWID required in 2016/2017 to reduce incidence to 2 per 100 pyr (2%) among PWID by 2026 with and without scale-up of OST and NSP to 80% coverage is shown in Fig. 5. In Amsterdam, an incidence of 2% (1-3%) was already estimated in 2016, and so just switching to DAAs ensured an incidence <2% by 2026 in 99% of model runs. In Slovenia, just switching to the new DAAs and maintaining the current treatment rates are likely to decrease incidence to <2% by 2026 (projected by 78% of model fits), with an increase in treatments rates by 20% being needed to ensure this impact in the other 22% of model fits. In Czech Republic, switching to DAAs would achieve 2% incidence in <10% of model fits. Increasing the current treatment rates by 43% over all model runs would ensure the decrease. In all other sites, a substantial increase in HCV treatment rates (in the absence of any increase in OST and NSP coverage) is needed to reduce HCV incidence to 2%. This ranges from three to five times the current treatment rates in France and Scotland, to between six and nine times in Hamburg, Norway, Denmark, Belgium, 17 times in Sweden, and 200 times in Finland. If OST and NSP are scaled-up to 80% coverage, maintaining the current treatment rates is sufficient to achieve an incidence of 2% in 2026 in Amsterdam and Slovenia (100% of model fits), may achieve this impact in Belgium and Czech Republic (84% and 50% of model fits), but is unlikely to (<10% of model fits) in other settings. Alongside increased OST and NSP, France, Denmark, Norway, Scotland, Hamburg, Sweden, and Finland would need to scale-up their baseline treatment rates by 2.2-, 2.8-, 2.8-, 3.6-, 4.7-, 10.3-, and 159-fold, respectively. This is 20-60% less than if OST and NSP had not been scaled-up.  
Uncertainty analysis  
The sensitivity analysis indicates that, for most sites, uncertainty in three main factors contributes to variation in the relative decrease in chronic prevalence and incidence between 2016 and 2026 when treatment rates are doubled, but with differing levels of influence between the sites (Fig. S4). The PWID population size contributes 34-63% of the variation in Finland, Belgium, Scotland, Slovenia, and Norway, and 80% in Sweden, whilst the prevalence estimates contribute 32-53% of the variation in five of the sites (Slovenia, Belgium, Czech Republic, Denmark, and Hamburg). The duration of injection is most important in Amsterdam, contributing 85% of the variation, but also contributes 25-48% in Scotland, Hamburg, Denmark, Norway, Belgium, and Finland. In France, the estimated treatment rate contributes 80% of the variation.  
Discussion  
Main findings  
Treatment scale-up is needed to achieve observable reductions in chronic HCV prevalence among PWID in most sites in Europe, even with new DAAs. Doubling the DAA treatment rates may lead to observable reductions (12-24% decrease) in chronic prevalence by 2026 in Belgium, Denmark, Hamburg, Norway, and Scotland; but not in Finland or Sweden. Exceptions include Czech Republic, Slovenia, and Amsterdam, which, at current HCV treatment rates, are projected to reduce chronic HCV prevalence from a third to a half by 2026. This is because of the low or decreasing prevalence of infection in these settings. Alternatively, increasing OST and NSP coverage to 80% with the current HCV treatment rates would reduce chronic HCV prevalence by 17-20% in Finland and Hamburg, and 30-79% in all other sites. Reducing HCV incidence to <2% by 2026 requires little action in Amsterdam, Czech Republic, and Slovenia, whereas in Belgium, Denmark, Hamburg, Norway, and Scotland it will require at least a fivefold increase in the current HCV treatment rates, or 1.8- to 4.7-fold if OST and NSP are scaled-up to 80% coverage.  
Strengths and limitations  
Our model projections and their interpretation are influenced strongly by uncertainty in the parameters and evidence base. First, we collected information from a range of sources and obtained data not routinely collected across Europe (e.g. number of PWID treated for HCV).[6], [28] Unfortunately, data collection was inconsistent across sites, particularly estimates of PWID population size, which were used to estimate HCV treatment rates. Reliable PWID population-size estimates are difficult to obtain. Except for Amsterdam where evidence suggests a falling population[15], [29] we had to assume stable populations.  
Second, uncertainty in the chronic HCV prevalence among PWID contributed substantially to the uncertainty in our projections, with estimates generated from a diverse range of sources and rarely (except for Scotland) from ongoing community-based surveillance.[6], [30]  
Third, the duration of injecting drug use is difficult to estimate precisely and contributed to model uncertainty. We sampled the average injecting duration from a range extending from 6 to over 20 years. In the absence of clear evidence, we also assumed that opioid and methamphetamine injectors had similar durations. If the true duration is towards the higher end of our ranges, scaling-up HCV treatment will have a greater impact on transmission. If towards the lower end, scaling-up OST and NSP will have a greater contribution on reducing transmission.10  
Fourth, DAA SVRs for PWID in "real world" settings are yet to emerge, and so we assumed a range of 85-95%.[31], [32] Given the short treatment duration and early treatment of a predominantly mild disease, it is likely that SVRs will be very high. However, it is possible that, as treatment is scaled-up among more vulnerable PWID, the SVR may reduce. In general, the impact of HCV treatment in our projections is relatively robust to variations in SVR. However, an uncertainty in SVR becomes more influential in settings with lower chronic prevalence and higher HCV treatment rates. Furthermore, we assumed that PWID who had either cleared HCV spontaneously or after successful treatment had the same risk of re-infection as the susceptible population of PWID i.e. the per capita transmission probability of re-infection was the same as for primary infection. There is some evidence to suggest that previous spontaneous clearance could result in higher rates of clearance for subsequent reinfection.22 However, similar data surrounding spontaneous clearance of reinfections after SVR do not exist. Infrequent testing intervals can contribute bias, as some reinfections may go unnoticed.[22], [33] Observational studies have reported that reinfection after SVR can be of a similar, higher, or lower rate than the background rate of infection,[34], [35], [36], [37], [38] indicating uncertainty in the evidence. However, if the reinfection risk was lower than the primary infection for people achieving SVR, or the spontaneous clearance is higher for reinfections, then our model projections represent conservative estimates for the number of treatments needed to reduce prevalence and incidence across the different sites.  
Fifth, we recorded substantial differences in coverage of OST and NSP between sites that are incorporated into the baseline model. In subsequent intervention scenarios, we either considered no scale-up of these interventions, or assumed their scale-up to 80% coverage. This optimistic scenario may overestimate the likely impact of what could be achieved from scaling-up OST and NSP, although some of our sites demonstrate such coverage is possible. However, even if this scale-up is possible, it is unlikely that it would be achieved quickly, and so these projections may overestimate the real reduction in HCV that could be achieved from scaling-up OST and NSP.  
Sixth, the model does not incorporate information on HCV case-finding and any future difficulty in diagnosing and treating PWID with chronic HCV when HCV transmission and prevalence have fallen to low levels. However, this limitation will only affect a small number of the most optimistic model projections.  
Seventh, we have not modelled HIV co-infection, which varies across Europe and may impact both on linkage to services and morbidity outcomes.  
Finally, we assume no change in injecting-risk behaviour following HCV treatment-apart from through exposure to OST and NSP, which is also included prior to HCV treatment. If injecting risk was reduced following treatment,39 our assumption provides conservative projections of impact.  
Implications and comparisons with other literature  
Multiple studies in specific countries and across Europe have used statistical and mathematical model projections to suggest that new DAA treatments need to increase to reverse trends in end-stage liver disease.[40], [41], [42], [43], [44] However, to project impact on HCV transmission, a dynamic transmission model that can track both reinfection and prevention of future infections is required, alongside information on the number and proportion of individuals from key populations, like PWID treated for HCV infection. Consequently, there are fewer analyses that project impact on HCV transmission.  
An earlier study revealed a two- to threefold difference in chronic HCV prevalence and four- to fivefold difference in baseline HCV treatment rates in seven cities in the UK.45 We also found considerable heterogeneity between sites in Europe. For example, the Czech Republic and Slovenia both have baseline chronic prevalence of <30%,[25], [46], [47] whilst in Finland and Sweden it is over 55%. Treatment rates also varied two- to threefold. At baseline, 8/11 sites had low treatment rates (<10/1,000 PWID treated annually), whereas France had a much higher treatment rate (21/1,000 PWID treated annually). This is a consequence of the high access to HCV treatment in France compared to other countries.41 In this study, a greater decrease in prevalence for Amsterdam is shown than was projected in other studies.[15], [48] However, this could be caused by differences in modelling the decreasing epidemic to achieve the incidence estimate, and differences modelling the PWID population and transmission dynamics.  
The lack of ongoing surveillance data, including PWID prevalence and HCV treatment rates among PWID, in many European settings and comparable indicators between countries is important and a public health concern. Using our model projections, it was shown that scaling-up OST and NSP combined with switching to DAAs with comparatively small increases in the number of PWID treated could generate substantial observable reductions in HCV prevalence in several sites. However, robust HCV surveillance data among PWID were not always available and chronic HCV prevalence was uncertain. To ensure that empirical evidence of the impact of HCV treatment as prevention can be generated, it is important that more attention is given to establishing robust surveillance systems to reduce the uncertainty surrounding chronic HCV prevalence among PWID. The potential and relative costs of introducing effective HCV surveillance are trivial compared to the costs of HCV treatment, and need to be encouraged across Europe.
| |
|
|
|
|
|