|
DDI + Tenofovir Interaction Study: no intracellular interaction, but high TDF levels after discontinuation
|
|
|
"Measurement of Intracellular Didanosine and Tenofovir Phosphorylated Metabolites and Possible Interaction of the Two Drugs in Human Immunodeficiency Virus-Infected Patients"
Antimicrobial Agents and Chemotherapy, May 2005, p. 1907-1914, Vol. 49, No. 5
.....Forty-three patients were included in the current trial; three of them participated in both the cross-sectional and the longitudinal studies..... To study the intracellular (IC) metabolism of ddI and TDF and their possible IC interaction, we measured ddA-TP (ddI) and TFV-DP (tenofovir) in PBMCs from patients receiving 250 mg of ddI plus 300 mg of TDF and compared the results with measurements in patients treated with only one of the two drugs.... Comparison of IC ddA-TP and TFV-DP levels in patients cotreated with ddI and TDF showed no significant difference with regard to the levels measured in patients treated with ddI alone or TDF alone.... Surprisingly, very significant levels of TFV-DP were measured in those patients 3 weeks after discontinuation of TDF, and the corresponding half-life of elimination was roughly calculated as 180 hours...in this study....it clearly appears that both ddI and TDF generate triphosphate metabolites with an unusually long half-life. This is certainly advantageous in terms of efficacy, because this ensures a constant and optimal IC level of the active drugs between two dosings and supports their use in QD regimens. However, this could have clinical consequences for patients discontinuing these drugs, because they can be exposed to suboptimal concentrations of the active triphosphates for a long period of time, with possible outcomes in terms of virus resistance......
authors: Alain Pruvost,1 Eugenia Negredo,2 Henri Benech,1 Frédéric Theodoro,1 Jordi Puig,2 Eulàlia Grau,2 Elisabet García,2 José Moltó,2 Jacques Grassi,1* and Bonaventura Clotet2
CEA, Service de Pharmacologie et d'Immunologie, DSV/DRM, CEA/Saclay, 91191 Gif sur Yvette cedex, France,1 Lluita contra la SIDA and "Irsicaixa" Foundations, Germans Trias i Pujol Hospital, Badalona, Barcelona, Spain2
INTRODUCTION
Nucleoside reverse transcriptase inhibitors (NRTIs) were the first antiretroviral class shown to be effective against human immunodeficiency virus (HIV) infection and still play a critical role as major components of highly active antiretroviral therapy. The first generation of NRTIs comprised 2',3'-dideoxynucleosides which have to undergo three phosphorylation reactions within cells and work both as competitive inhibitors and as chain terminators with regards to HIV reverse transcriptase.
Closely related drugs, i.e., acyclic phosphonate analogues of nucleotides (NtRTIs), have recently been approved for clinical applications and are now given as part of combination antiretroviral regimens. This new strategy consists of directly providing the cell with the monophosphate analogue, thus bypassing the first phosphorylation step, which proved to be rate limiting for many NRTIs. The first approved drug of this category is tenofovir disoproxyl fumarate (TDF), which provides high intracellular (IC) levels of tenofovir diphosphate (TFV-DP, equivalent to NRTI triphosphate) with very low mitochondrial toxicity (7).
However, the issue of drug-drug interactions between NRTIs and also between NRTIs and NtRTIs is of major concern and should be addressed with all the analytical and chemical tools available. NRTIs and NtRTIs might interact not only at the systemic level (absorption, metabolism, distribution, or elimination) but also at the cellular level through membrane transfer or IC metabolism. All these drugs undergo a very complex IC metabolism dependent on IC kinases which are subject to competition and inhibition, or whose turnover may be limited. Several IC interactions have been found, in vitro and in vivo, between NRTIs sharing the same metabolic pathways, i.e., between zidovudine and stavudine for thymidine analogues (10), which are clinically relevant (9), and lamivudine (3TC) and zalcitabine for cytidine analogues (11).
Recently, a significant interaction between didanosine (ddI) and TDF leading to an increase in plasma concentrations of ddI has been clinically demonstrated (8, 13). Recent reports reviewed elsewhere (16) have shown that when healthy subjects are given didanosine buffered tablets followed 1 hour later by TDF, for 7 days, the mean area under the curve (AUC) for plasma didanosine concentrations was 44% higher than when didanosine was given alone. A similar study examined enteric-coated didanosine given alone or with TDF, and the didanosine AUC was again increased by 48% when given 2 hours prior to TDF and a light meal and by 60% when given simultaneously with TDF and a light meal (B. P. Kearney, A. Plummer, J. Sayre, X. Zhang, A. K. Cheng, K. Ryan, and B. Damle, Abstr. 14th Int. AIDS Conf., abstr. LbPeB9026, 2002). The mechanism underlying this interaction is not known, but recently it was hypothesized that this could result from inhibition of the cellular enzyme purine nucleotide phosphorylase by TDF mono- and diphosphate (18). Since purine nucleotide phosphorylase plays a critical role in the catabolism of purine nucleoside, its inhibition may result in an increase in plasma ddI concentration. It is worth noting that the ddI-TDF interaction may have a clinical impact, since two recent papers reported an unexpected and significant CD4 decline in patients receiving both ddI (400 mg, once a day [QD]) and TDF (300 mg, QD) (14, 15). However, the mechanism of this cellular toxicity has not been elucidated. In addition, pancreatic toxic effects have been associated with coadministration of ddI and TDF (12).
Interestingly, when the didanosine dosage was reduced to 250 mg, the plasma didanosine AUC reached the levels obtained when 400 mg of didanosine was given alone (i.e., without TDF). To date, the recommended didanosine dose given with TDF (300 mg, QD) is 250 mg QD for patients weighing >60 kg and 125 to 200 mg for those weighing <60 kg, instead of 400 mg and 250 mg, respectively, in the absence of TDF (B. P. Kearney, E. Isaacson, J. Sayre, H. Namini, and A. Cheng, Abstr. 10th Conf. Retroviruses Opportunistic Infect., abstr. 533, 2003; S. Kaul, B. Damle, K. Bassi, J. Xie, J. Gale, K. Ryan, and G. Hanna, Abstr. 4th Int. Work. Clin. Pharmacol. HIV Ther., abstr. 54, 2003).
As ddI and TDF are both nucleoside and nucleotide analogues of adenosine, their pharmacological IC interaction should also be considered, because they partly share the same IC metabolic pathway. The IC metabolism of ddI leads first to ddI monophosphate (ddI-MP) via a 5'-nucleotidase acting as inosine phosphotransferase. In a second step, ddI-MP is converted to dideoxy adenosine-MP (ddA-MP) by the combined action of adenylosuccinate synthetase and adenylosuccinate lyase. In a third step, ddA-MP is transformed into ddA-DP by adenyl kinase, before the third phosphorylation step catalyzed by the ubiquitous nucleotide diphosphate kinase takes place to produce ddA triphosphate (ddA-TP) (1). On the other hand, the IC metabolism of tenofovir leads, after two phosphorylation steps, to the formation of the active form (TFV-DP) by using successively adenyl kinase and nucleotide diphosphate kinase, the last two enzymes involved in the production of ddA-TP (19).
Recently it has been shown in in vitro experiments with cultured cells that the IC metabolism of didanosine is not modified by tenofovir and vice versa (20). It is worth noting that most available data on the IC metabolism of ddI and TDF come from in vitro experiments because of the difficulty of measuring IC concentrations of NRTI and NtRTI phosphates. In order to improve our knowledge on this IC behavior of ddI and TDF phosphates and to clarify the consequences of the coadministration of the two drugs in clinical situations, we have analyzed IC ddA-TP and TFV-DP contents in peripheral blood mononuclear cells (PBMCs) from patients receiving ddI or TDF alone in comparison with those from cotreated patients by using validated liquid chromatography-tandem mass spectrometry (MS/MS) techniques (3, 4) applied to TFV-DP measurements.
ABSTRACT
Recent work has demonstrated the existence of a systemic interaction between didanosine (ddI) and tenofovir disoproxyl fumarate (TDF) that leads to a significant increase in plasma ddI levels when coadministered with TDF (40 to 50% increase).
These two drugs are, respectively, nucleoside and nucleotide analogues of adenosine and efficiently inhibit the human immunodeficiency virus (HIV) reverse transcriptase when transformed to their triphosphate moieties in the intracellular (IC) medium (ddA-TP and TFV-DP, respectively).
Since ddI and TDF partly share the same IC metabolic pathway leading to the active triphosphates, we investigated a putative IC interaction. We used high-performance liquid chromatography-tandem mass spectrometry techniques to determine ddA-TP and TFV-DP IC levels in HIV-infected patients cotreated with both drugs, in comparison with patients treated with just one of the two drugs.
These measurements revealed no significant differences in IC levels of the corresponding triphosphates when ddI (250 mg, once a day [QD]) was coadministered with TDF (300 mg, QD) compared to ddI 400 mg (QD) administered without TDF, thus supporting the dose adaptation proposed for this combination.
..... It is worth noting, however, that the results recorded during this study do not allow us to conclude that there is absolutely no IC interaction between ddI and TDF. In fact, a close examination of the data reveals a tendency (P values of 0.170 and 0.150 are close to significance) to a lowering of IC concentrations of both ddA-TP and TFV-DP when the two drugs are used in combination. Clearly, the variations are too small to be revealed in this kind of cross-sectional study, due to interindividual variations, but could possibly be demonstrated in longitudinal studies.....
However, we observed that both ddA-TP and TFV-DP have very long IC half-lives, resulting in unusual IC pharmacokinetic profiles with no significant changes in triphosphate concentrations between two dosings.
In the case of TFV-DP, this t1/2 of elimination was roughly estimated to be 180 h (7.5 days). This characteristic is certainly interesting in terms of efficacy but could have some drawbacks in terms of virus resistance for patients discontinuing these drugs.
.... Surprisingly, very significant levels of TFV-DP were measured in those patients 3 weeks after discontinuation of TDF (Table 2; see table at end of this report), and the corresponding half-life of elimination was roughly calculated as 180 h (7.5 days)....
.... Taking into account the small number of patients included in this discontinuation study, with only two time points over a 3-week interval, this value has to be measured more precisely, but it is clearly the longest half-life measured for an NRTI or NtRTI. The first consequence of this unusually long half-life is that possible selection of resistance mutations after discontinuation of TDF in standard therapeutic interruptions should be addressed, since cells can be exposed to suboptimal doses of TFV-DP over a long period of time, as already suggested for nonnucleoside RTIs...
......it clearly appears that both ddI and TDF generate triphosphate metabolites with an unusually long half-life. This is certainly advantageous in terms of efficacy, because this ensures a constant and optimal IC level of the active drugs between two dosings and supports their use in QD regimens. However, this could have clinical consequences for patients discontinuing these drugs, because they can be exposed to suboptimal concentrations of the active triphosphates for a long period of time, with possible outcomes in terms of virus resistance. (see tables at end of this report).
RESULTS
Forty-three patients were included in the current trial; three of them participated in both the cross-sectional and the longitudinal studies. Most participants were men (16% women), and the mean age was 38 years (standard deviation [SD], ±11), with no differences between treatment groups. The clinical status of most patients (81%) was good (A or B classification according to the Centers for Disease Control and Prevention stage of HIV infection), and the mean CD4 cell count was 554 cells/mm3 (SD, ±273). On entering the study, none of the participants presented an acute infection or other opportunistic disease. None had renal dysfunction (defined as increases of plasma creatinine or blood urea nitrogen 2.5 or 1.5 times the normal value, respectively [grade II] or hypophosphatemia of <0.62 mmol/liter [grade II]) that could have influenced the pharmacokinetic parameters analyzed.
To study the IC metabolism of ddI and TDF and their possible IC interaction, we measured ddA-TP and TFV-DP in PBMCs from patients receiving 250 mg of ddI plus 300 mg of TDF and compared the results with measurements in patients treated with only one of the two drugs. IC measurements are listed in Table 1. The mean IC ddA-TP value measured in patients of group 2 (ddI alone) was 7.83 fmol/106 cells (median, 6.70 fmol/106 cells). The mean TFV-DP level in patients of group 1 (TDF alone) was 190.5 fmol/106 cells (median, 158.4 fmol/106 cells). For both ddA-TP and TFV-DP, time course measurements (when available showed no significant variation in IC levels between baseline and other sampling times (up to 4 hours after dosing).
For TFV-DP this is also shown, in determinations performed on the series of five patients during a 12-hour kinetics study. These data were used to calculate interindividual variations in concentrations, which ranged from 42 to 82% for groups 1 and 3.
Comparison of IC ddA-TP (mean, 6.74 fmol/106 cells; median, 5.06 fmol/106 cells) and TFV-DP (mean, 192.6 fmol/106 cells; median, 134.5 fmol/106 cells) levels in patients cotreated with ddI and TDF showed no significant difference with regard to the levels measured in patients treated with ddI alone (mean, 7.83 fmol/106 cells; median, 6.70 fmol/106 cells; P = 0.170) or TDF alone (mean, 190.5 fmol/106 cells; median, 158.4 fmol/106 cells; P = 0.152). This was also supported by measurements of IC ddA-TP and TFV-DP in patients on ddI (250 mg, QD) plus TDF (300 mg, QD) plus NVP (400 mg, QD) for several months who switched to 3TC (300 mg, QD) plus ddI (400 mg, QD) plus NVP (400 mg, QD). Here again, there was no significant difference in IC ddA-TP levels before and after the discontinuation of TDF and the switch (mean, 13.8 fmol/106 cell versus 11.3 fmol/106 cells). Surprisingly, very significant levels of TFV-DP were measured in those patients 3 weeks after discontinuation of TDF, and the corresponding half-life of elimination was roughly calculated as 180 h (7.5 days).
AUTHOR DISCUSSION
The ideal approach to studying the effect of TDF on ddI IC metabolism, in relation to the plasma interaction, would have been to measure IC ddA-TP levels in patients already on ddI therapy (400 mg, QD) and starting a new TDF-containing regimen. The influence of a decrease in ddI dosage from 400 mg to 250 mg on the IC ddA-TP concentrations could have been evaluated in a second step. Unfortunately, at the time when this study was initiated, the recommendation had already been made for dosing patients on combined ddI plus TDF therapy with 250 mg of ddI, because of severe toxicity problems, and such a study was not ethically justified. Instead of a longitudinal study we then changed to a cross-sectional study, looking at ddA-TP and TFV-DP IC levels in patients on 250 mg ddI plus 300 mg TDF compared with levels in patients treated with only one of the two drugs. As far as possible, the patients on TDF were selected so that their regimen did not include any other drug suspected to interact with TDF, and so patients on lopinavir-ritonavir were excluded from this study (see Materials and Methods).
IC triphosphates of ddI (ddA-TP) and diphosphates of tenofovir (TFV-DP) were quantified at the IC level by using fully validated (3) liquid chromatography coupled with tandem mass spectrometry (4), which is one the most specific and sensitive techniques available to pharmacologists. Among other advantages, it allows the simultaneous quantification of several drugs, such as TFV-DP and ddA-TP, in the same analysis, thus lowering the variability of the assay. This method has already been applied to clinical samples for the quantification of IC metabolites of NRTIs such as stavudine (21), stavudine and didanosine (2), and zidovudine (5).
The assessment of IC TFV-DP concentrations in our study is among the first with a large number of patients treated with the usual dosage of TDF (300 mg, QD). Average and median values for IC TFV-DP were in the range of 130 to 200 fmol/106 cells, confirming that TDF therapy delivers high concentrations of TFV-DP within the cells. Interindividual variations (ranging between 42 and 82%) were also similar to those recorded in previous studies for ddA-TP or stavudine-TP (58 and 48%, respectively) (2). An important observation is that, at steady state, no significant variation in IC TFV-DP concentrations was seen between two successive dosings. This suggests that the t1/2 of elimination of TFV-DP is much longer than the time separating two dosings and cannot be calculated from concentration data collected over 24 h. This is in agreement with previous measurements performed in vitro, which showed t1/2 values of elimination of up to 15 and 50 h in activated and resting lymphocytes, respectively (19). This is also supported by the results of a recent clinical study on HIV-infected patients, which showed a t1/2 of elimination above 60 h (T. Hawkins, W. Veikley, R. St. Claire, A. Hey, B. Guyer, and B. P. Kearney, Abstr. 5th Int. Work. Clin. Pharmacol. HIV Ther., abstr. 6, 2004). TFV-DP half-life can be estimated in patients discontinuing TDF after several days or weeks. In this study, data collected on three patients suggest an approximate t1/2 of elimination of 180 h (7.5 days). Taking into account the small number of patients included in this discontinuation study, with only two time points over a 3-week interval, this value has to be measured more precisely, but it is clearly the longest half-life measured for an NRTI or NtRTI. The first consequence of this unusually long half-life is that possible selection of resistance mutations after discontinuation of TDF in standard therapeutic interruptions should be addressed, since cells can be exposed to suboptimal doses of TFV-DP over a long period of time, as already suggested for nonnucleoside RTIs (S. Taylor, S. Allen, S. Fidler, D. White, S. Gibbons, J. Fox, J. Clarke, J. Weber, P. Cane, A. Wade, E. Smit, and D. Back, Abstr. 11th Conf. Retroviruses Opportunistic Infect., abstr. 131, 2004). In addition, one would expect that the time to steady state would also be very long.
The same observation holds for ddA-TP, for which we were unable to calculate any half-life in the absence of discontinuation studies. However, in vitro experiments also suggest a long half-life of elimination of 40 h (1), which is far too long to be derived from measurements performed between two dosings in patients (2).
IC ddA-TP concentrations reached when didanosine was given without TDF were in agreement with those previously published (2) (median, 6.7 versus 8 fmol/106 cells). When didanosine was given at 250 mg QD together with TDF at 300 mg QD, IC ddA-TP concentrations were not statistically different from those reached without TDF (median, 5.06 versus 6.70 fmol/106 cells; P = 0.170). This suggests that the dosing adaptation proposed for the combined use of TDF and ddI, i.e., switching from 400 mg QD to 250 mg QD (for patients weighing >60 kg), is appropriate and that observed changes in plasma ddI levels are reflected at the IC level. We have no information concerning IC levels of ddA-TP when ddI is used at a 400-mg QD dose simultaneously with TDF, since the corresponding samples were no longer available, for ethical reasons, when this study was initiated. Nevertheless, our results do not suggest that under these conditions unusually high IC levels of ddA-TP are produced, which could explain the cellular toxicity reported for the ddI (400 mg, QD) plus TDF (300 mg, QD) combination (12, 14, 15).
It is worth noting, however, that the results recorded during this study do not allow us to conclude that there is absolutely no IC interaction between ddI and TDF. In fact, a close examination of the data (Table 1) reveals a tendency (P values of 0.170 and 0.150 are close to significance) to a lowering of IC concentrations of both ddA-TP and TFV-DP when the two drugs are used in combination. Clearly, the variations are too small to be revealed in this kind of cross-sectional study, due to interindividual variations, but could possibly be demonstrated in longitudinal studies.
In conclusion, this study does not support the existence of a strong IC interaction between ddI and TDF and shows that the dose adaptation proposed on the basis of plasma assays is appropriate. In addition, it clearly appears that both ddI and TDF generate triphosphate metabolites with an unusually long half-life. This is certainly advantageous in terms of efficacy, because this ensures a constant and optimal IC level of the active drugs between two dosings and supports their use in QD regimens. However, this could have clinical consequences for patients discontinuing these drugs, because they can be exposed to suboptimal concentrations of the active triphosphates for a long period of time, with possible outcomes in terms of virus resistance.
MATERIALS and METHODS
Patients. The study was proposed to subjects who had been receiving a triple highly active antiretroviral therapeutic regimen for more than 24 weeks that included ddI and/or TDF, both administered in the morning, who were consecutively seen between April 2003 and March 2004 in the HIV Unit of Germans Trias i Pujol Hospital (Barcelona, Spain). Subjects who accepted were included in the study and classified based on NRTI combination (see below).
Patients treated with lopinavir-ritonavir were not included in the study, because this protease inhibitor has been reported to interact with TDF (http://www.thebody.com/confs/icaac2003/pdfs/A-1617_poster.pdf). In addition, other exclusion criteria were a suspected improper drug adherence or treatment discontinuation during the previous 3 months.
Cross-sectional study. Groups in the cross-sectional study were as follows. Group 1 (n = 8) included patients on TDF (300 mg, QD, with food) and not treated with ddI. Most of them were cotreated with 3TC and nevirapine (NVP). Group 2 (n = 16) consisted of patients on ddI (400 mg, QD, without food) and not treated with TDF. Most of them were cotreated with 3TC and NVP. Group 3 (n = 14) included patients on TDF (300 mg, QD) and ddI (250 mg, QD) and all were cotreated with NVP at breakfast time. Blood samples were taken at baseline (just before dosing) and during the 4 hours following dosing.
Kinetic study. Five patients on TDF (300 mg, QD) plus ddI (250 mg, QD) plus NVP for more than 24 weeks were sampled at dosing and 1, 2, 4, 6, 8, 10, and 12 h after dosing. In this series, only TFV-DP was determined.
Longitudinal study in patients discontinuing TDF. Three of the patients who had been on ddI (250 mg, QD), TDF (300 mg, QD), and NVP for more than 24 weeks were switched to a regimen that replaced TDF with 3TC and with ddI increased to 400 mg, QD. Samples were taken 2 hours after the last dosage of the combination, while measurements performed after 3 weeks of TDF interruption were made at baseline, 2, and 4 h after dosing.
Materials and chemicals. CPT tubes (catalog no. 362761; Becton Dickinson, Franklin Lakes, N.J.) were used for separation of PBMCs from blood. ddA-triphosphate (2',3'-dideoxyadenosine-triphosphate) was from Amersham Pharmacia Biotech. TFV-DP (9-R-[2-phosphonomethoxypropyl]adenine diphosphate) was from Gilead Sciences (Foster City, Calif.). Cl-ATP (2-chloroadenosine 5'-triphosphate), Ficoll Histopaque-1077, formic acid (ammonium salt), and 1,5-dimethylhexylamine were from Sigma-Aldrich (St. Quentin-Fallavier, France). Analytical formic acid was from Merck (Darmstadt, Germany). Analytical acetonitrile was from S.D.S. (Peypin, France). Blood was from the French Blood Transfusion Service (EFS; Rungis, France). Nitrogen HP45 was from Air Liquide (Paris, France). A 0.9% NaCl solution was prepared in ultrapure water.
Preparation of stock and diluted solutions. ddA-TP was provided in a 100 mM solution (pH 7.0). Stock solutions of ddA-TP, TFV-DP, and an internal standard (concentration, ~100 µg/ml) were prepared in ultrapure water and stored at around -20°C. Diluted intermediate solutions of ddA-TP and TFV-DP (concentration, ~10 µg/ml and then ~1 µg/ml) were prepared by serial dilution of stock solutions in ultrapure water and were stored at around -20°C. The working solutions of ddA-TP and TFV-DP (concentration, ~100 ng/ml) were prepared each day in ultrapure water and were stored at around 4°C. Standards and quality controls were prepared from these solutions. The working solutions of internal standard were prepared daily from an intermediate solution by addition of 1,500 µl of ultrapure water to obtain a final concentration around 160 ng/ml.
Analytical methodology. The assay of IC ddA-TP has been previously described (4). For the simultaneous determination of ddA-TP and TFV-DP, the liquid chromatography part was exactly the same as previously described (4, 16). Briefly, the chromatographic separation was achieved on a Supelcogel ODP 50, 5-µm (150- by 2.1-mm) column (Sigma-Aldrich-Supelco), thermostat at 30°C, with a mobile phase comprising an ion-pairing reagent (dimethylhexylamine) mixed with an ammonium formate buffer and an acetonitrile gradient delivered at a flow rate of 0.3 ml/min. A new mass spectrometer was used (triple quadrupole tandem mass spectrometer; Quantum Discovery with an ESI source from Thermo-Electron) monitored via data acquisition and treatment software Excalibur version 1.3. The assay for TFV-DP was fully validated with this mass spectrometer, and a partial validation was performed for ddA-TP regarding especially the new lower limit of quantitation (LOQ) and the matrix effect according to the number of cells. The new tube parameters, optimized for the ionization and detection of both compounds, were as follows. The spray voltage was -3.3 kV. The values of the tube lens offset were -99 V, -108 V, and -133 V for TFV-DP, ddA-TP, and internal standard, respectively. Nitrogen was used for spraying (flow, 0.74 l/min) and auxiliary (auxiliary flow, 4.5 l/min) gas. The capillary temperature was set at 330°C. The instrument was operated in the electrospray negative mode under MS/MS conditions. Fragmentation was achieved with argon (collision gas pressure, 1.5 mTorr). Collision energy was 28 V for ddATP and 36 V for both TFV-DP and the internal standard. The ion transitions monitored were m/z 474->159 for ddA-TP, 446->159 for TFV-DP, and 540->159 for the internal standard. Dwell time was 0.5 s for each transition. Retention times were around 10.8 min for ddA-TP and TFV-DP and 11.8 min for the internal standard. Under these conditions, the lower LOQ for the ddA-TP measurement was improved and the new value was found to be 25 fmol/sample instead of 53.4 fmol/sample. For this purpose, three experiments were conducted on six different samples analyzed in the same run, and the mean precision and accuracy were 17.6% and 10.3%, respectively. On the other hand, since the ionization recovery (matrix effect) is mass spectrometer related, ionization recovery was studied on the TSQ Quantum apparatus with various amounts of PBMCs at the lowest quality control amount (80 fmol) on the ddA-TP/internal standard ratio. The ionization recovery, ddA-TP/internal standard ratio, was constant whatever the cell count was, between 3 x 106 cells and 21 x 106 cells. With respect to the assay of TFV-DP, interrun precision and accuracy at the lower LOQ (106 fmol/sample) were 11.4% and 108.3%, respectively, and intrarun precision and accuracy at this level were 8.9% and 114.1%, respectively. Intra- and interrun precision and also accuracy were checked with quality control samples containing TFV-DP at three concentrations covering the range of the calibration curve. Interrun precision and accuracy ranged from 6.4 to 14.0%, and mean accuracy ranged from 97.0 to 100.1%. Intrarun precision and accuracy ranged from 7.1 to 12.9%, and mean accuracy ranged from 91.3 to 113.8%. The same experiments were performed for TFV-DP regarding the matrix effect as described above for ddA-TP, and the same result was obtained.
PBMC preparation for standards and QCs. Approximately 8 ml of blood was transferred to Becton Dickinson CPT (cell preparation tube) Vacutainer tubes containing sodium citrate as anticoagulant and centrifuged at room temperature (18 to 25°C) for 25 min at 1,500 to 1,800 x g as previously described (4, 17). After centrifugation, the PBMCs were washed twice using 0.9% NaCl solution at 4°C. Samples containing between 7 x 106 and 10 x 106 cells/tube were collected and stored at -80°C for further utilization for standards and quality controls (QCs).
Sample treatment. Standards containing both TFV-DP and ddA-TP were prepared as follows: 20 µl of internal standard solution (~160 ng/ml) was added to one pellet of blank PBMCs stored at -80°C, followed by 20 µl of the compounds of interest mixed in solution at an adjusted concentration. Final amounts of TFV-DP (and ddA-TP) were 106 (25), 212 (50), 424 (712), 1,060 (1,424), 1,908 (1,960), and 2,968 (2,672) fmol/sample containing around 7 x 106 to 10 x 106 cells.
QCs were usually prepared as a pool and stored with the samples. Since a sufficient amount of blood was not available to prepare all the necessary QCs for the study, they were prepared on each day of analysis in triplicate and as follows: 20 µl of internal standard solution (~160 ng/ml, around 4°C) was added to one pellet of PBMCs stored at around -80°C, followed by 20 µl of the compounds of interest in mixed solution (stored at around 4°C) in order to obtain final amounts of 254.5 (80), 1,484 (1,176), and 2,120 (2,136) fmol/sample containing around 7 x 106 to 10 x 106 cells.
Five hundred microliters of a mixture of Tris-HCl (0.05 M; pH 7.4)-methanol 30/70 (vol/vol) was added to the samples, standards, and QCs. Cells were mechanically lysed, and the solution was centrifuged (18,000 x g for 30 min at +4°C). The supernatants were immediately transferred into polypropylene tubes, and methanol was evaporated with a TurboVap evaporator (Zymark, France) for 30 min at 35°C. A 40-µl fraction of the remaining solution was injected into the high-performance liquid chromatography-MS/MS apparatus.
Clinical subject samples. PBMCs collected from 7 to 8 ml of blood from TDF- and/or ddI-treated patients were kept frozen at -80°C. PBMCs were transferred on dry ice to the analytical laboratory. PBMCs were spiked with 20 µl of internal standard (~160 ng/ml, around 4°C) and submitted to the same treatment as previously described for spiked samples.
Cell counting. The PBMCs of each clinical sample were counted in our laboratory using a validated biochemical test as previously described (6).
Statistical analysis. For management of values below the LOQ, in order to avoid rejecting values when they were below the lower LOQ, because of their weight and importance in case of group comparisons we applied the following rule (often used in similar cases and in pharmacokinetics). When the result for a PBMC sample was found to be below the lower LOQ, the value used was LOQ/2 (i.e., 12.5 fmol) divided by the number of cells to obtain the concentration in fmol/106 cells.
SigmaStat 3.0 (Sysstat Software Inc.) was used to perform statistical analysis to highlight any statistically significant difference between the data groups. One-way analysis of variance (Kruskal-Wallis one-way analysis of variance on ranks when normality test failed) was performed.
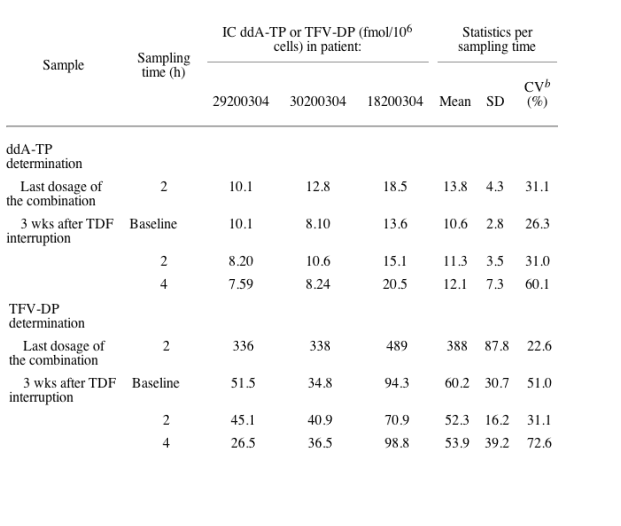
|
|
|
|
|
|
|