|
Metabolic bone disease in HIV infection: HIV, ART Implicated EDITORIAL
|
|
|
AIDS:
17 July 2009
Borderi, Marco; Gibellini, Davide; Vescini, Fabio; De Crignis, Elisa; Cimatti, Laura; Biagetti, Carlo; Tampellini, Livia; Re, Maria C
aSection of Infectious Diseases, Department of Internal Medicine, Geriatric Medicine and Nephrology, University of Bologna, Bologna, Italy
bDepartment of Haematology and Oncologic Sciences, Section of Microbiology, Italy
cCenter for Metabolic Bone Diseases, Gorizia Hospital, Gorizia, Italy dInteruniversity Consortium, National Institute Biostructure and Biosystem (INBB), Rome, Italy.
Conclusion: Bone derangement is a major clinical complication in the course of HIV infection [135]. The advent of HAART has led to a longer life expectancy and therefore HIV/HAART-related bone disease is destined to increase, enhancing the physiological age-related bone loss. Some epidemiological surveys in HIV-uninfected individuals indicate that the percentage of osteoporosis after 45 years of age ranges between 10 and 20%, and the lifetime fracture risk for a 50-year-old White woman is greater than 50% [136,137]. These data suggest that the number of HIV patients with bone disease and silent fractures can be expected to increase dramatically in the next few years because these patients also have two other potentially worsening factors: HIV itself and antiretroviral therapy. Hence, antiretroviral therapy must be accompanied by the clinical management of HIV/HAART-related bone disease to reduce the risk of osteoporosis and fractures in these patients.
a major effort should be made to obtain at least one BMD measurement in all HIV-infected patients......DXA should be performed in all patients, at least at baseline visit, as many prospective studies have demonstrated that it will predict fracture risk....low calcium diet has been demonstrated to reduce BMD and maybe to increase the hip fracture risk....cholecalciferol should be administered to all HIV-infected patients presenting vitamin D deficiency.
The determination of these cytokines is not yet part of the routine evaluation of HIV-infected patients.......Bone biology parameters can be monitored by laboratory tests: calcaemia, phosphataemia and albuminaemia (for ionized calcium calculation), urinary calcium and phosphate excretion. In addition, bone formation markers (i.e. osteocalcin or bone-associated alkaline phosphatase) and bone resorption markers (pyridinoline cross links or CTx, or NTx) can yield useful information together with vitamin D and PTH assays. Important information may be obtained by GFR determination (age and TDF, together with a low GFR, inducing a decrease in the function of alpha-1-hydroxylase in the kidney and in vitamin D availability) and the evaluation of kidney tubular function (some proximal tubular alterations may occur with normal GFR, and may signify an initial impairment of tubular function potentially unsafe for bone).
In addition to the direct effect of HIV replication, the apoptosis process plays a pivotal role in HIV pathogenesis......NRTIs inhibit the DNA polymerase-γ [75], the enzyme involved in the replication of mitochondrial DNA, leading to mitochondrial damage and dysfunction [76]. In-vitro studies disclosed some differences in the induction of specific NRTI-related mitochondrial DNA depletion. The so-called 'd-drugs' ddC (zalcitabine), ddI (didanosine), and d4T (stavudine) are relatively stronger inhibitors of polymerase-γ than other nucleoside analogues, called 'non-D drugs'......These data suggest that lactic acidaemia induced by NRTIs may cause osteopenia by a mechanism related to calcium hydroxyapatite loss as the bone tries to buffer chronic acidosis. This damage mainly affects the trabecular bone, which represents the majority of vertebral bone and is a more labile store of calcium than cortical bone. In addition the systemic effects of NRTIs, a paper by Pan et al. (Table 3) showed a specific interaction between zidovudine (ZDV) and bone. ZDV enhances the RANKL-mediated osteoclastogenesis inducing osteopenia in a murine model [81]......The impaired phosphorus balance and vitamin D metabolism related to renal toxicity may determine an osteomalacic pattern in HIV patients: some studies found an association between use of TDF and bone damage [97,98] and a higher incidence of foot fracture was also found in TDF-treated patients compared with TDF-untreated individuals [99,100].....Altogether these in-vitro studies suggest that some protease inhibitors may determine bone loss by increasing osteoclast resorption and inhibiting the osteoblast rebuilding function......Hypovitaminosis D was observed before the advent of HAART and a severe depletion of vitamin D was associated with advanced infection and immune system hyperactivation [117]. Recent studies performed on a cohort of naive and HAART-treated patients demonstrated a high prevalence of hypovitaminosis D suggesting a risk of osteomalacia
The management of osteopenia/osteoporosis in the course of HIV infection may be based on a change in risk factors, calcium and vitamin D diet supplementation and biphosphonate drugs (Table 5).....Patients can be advised to stop smoking and take physical exercise to control body weight. In addition, patients should have correct diet indications to ensure an adequate uptake of calcium and vitamin D. However, cholecalciferol should be administered to all HIV-infected patients presenting vitamin D deficiency.
Introduction
HIV mainly replicates in CD4+ T lymphocytes and monocyte/macrophages causing severe immunological impairment. In addition to the immune system, HIV infection affects tissues and organs such as kidney, liver, the central nervous system, heart and bone showing a complex pathogenesis [1].
The advent and widespread use of highly active antiretroviral therapy (HAART) in the last two decades has led to a marked improvement in the treatment of HIV disease even though viral infection cannot be eradicated because HAART does not completely eliminate the viral reservoirs [2]. HAART has dramatically changed the course of HIV infection from a fatal infection to a chronic and relatively manageable disease. The increased life expectancy of HIV patients and the effects of HAART have changed the management of HIV infection. Nowadays medical treatment is no longer focused solely on HIV infection, opportunistic diseases and monitoring immune derangement, but also includes the control of metabolic, cardiovascular, liver, bone and kidney complications. In particular, bone alterations have been observed in the course of HIV disease representing a pivotal clinical problem in the management of HIV patients especially for a possible development of bone fractures [3]. The major bone lesions detectable in HIV patients are related to bone demineralization (osteopenia/osteoporosis and osteomalacia) and osteonecrosis ([4] for a review).
This report will discuss the pathogenesis, diagnosis and treatment of major bone complications represented by bone demineralization diseases during HIV infection and HAART treatment.
Osteopenia/osteoporosis in HIV-infected patients
Bone alterations have been observed in the course of HIV disease since for nearly two decades (Table 1). In particular, reduced bone mineral density (BMD) is the most common bone lesion found in HIV-infected individuals [5,6]. BMD is a parameter that predicts fracture risk, which in turn correlates with a shorter life expectancy [7]. BMD is measured by the dual X-ray absorptiometry scan (DXA). According to the WHO Classification, BMD is commonly reported in terms of DXA T-score, which represents the number of standard deviations below the mean of a young, sex-matched control population. T-score values are considered normal above the limit of -1. Values between -1 and -2.5 indicate osteopenia (low bone mass) whereas a T-score value below -2.5 signifies osteoporosis [8,9]. Osteoporosis is a systemic condition characterized by both quantitative and qualitative alterations that reduce bone strength [10].
Several groups have used DXA to study BMD status during HIV infection. A meta-analysis of selected reports on bone loss in the whole HIV patient population (HAART treated plus naive) from 1994 to 2005 showed that these individuals had 6.4 fold increased odds of osteopenia and 3.7-fold increased odds of osteoporosis in comparison with uninfected individuals [11]. The relation between antiretroviral treatment and osteopenia/osteoporosis has been noted in several studies [12-18] although other reports failed to find any influence of HAART on bone loss, disclosing no major differences between naive and HAART treated patients [19-23]. A recent study on 492 patients belonging to the Aquitaine Cohort reported osteopenia in 50% and osteoporosis in 30% of HIV-positive cases but multivariate analysis did not show a significant correlation to bone loss and cumulative HAART or specific drug class [24].
In spite of these opposing findings, a meta-analysis of selected cross-sectional studies demonstrated that the odds of osteoporosis were increased 2.4 times in HAART-treated patients compared with naïve individuals [11]. In addition, the meta-analysis by Brown and Qaqish on 12 studies disclosed that patients treated with protease inhibitors have a higher prevalence of reduced BMD and the odds of osteoporosis in protease inhibitor-treated patients are 1.6 greater than in protease inhibitor-untreated individuals [11]. The controversy over the role of antiretroviral compounds in BMD decrease could be explained by shortcomings in some studies. HAART typically combines nucleoside analogue reverse transcriptase inhibitors (NRTIs) with either HIV protease inhibitors or nonnucleoside reverse transcriptase inhibitors (NNRTIs), thus, the antiretroviral cocktail composition may differ within the same cohort with conceivably different effects on bone. In addition, some DXA studies analysed only the spine (mainly confined to trabecular bone), or hip (mostly cortical bone) or both bone sites. The choice of bone for DXA assay is not negligible; the human skeleton is composed of two different types of bone tissue: trabecular bone (comprising around 20% of the bone and mainly involved in the maintenance of mineral homeostasis) and cortical bone (80% and responsible for most support functions). Plainly, high bone turnover states, such as HIV-induced osteoporosis, involve trabecular bone (spine) earlier and to a greater extent compromising cortical bone (hip) only much later [25]. Moreover, the effectiveness and duration of HAART treatment may also affect bone biology and hence the interaction between HAART and bone is noteworthy [26,27].
Mechanisms of HIV-associated osteopenia/osteoporosis
The pathogenesis of reduced BMD in HIV-infected patients is probably multifactorial. Osteopenia and osteoporosis are bone lesions mainly correlated to risk factors such as sex, age, low body weight, malnutrition, immobility, lifestyle factors (smoking, alcohol abuse), glucocorticoid, hypogonadism and lipodystrophy [28]. The sum of traditional patient-related risk factors with HIV infection and HAART side effects can determine the onset of these bone lesions in HIV-infected patients.
Bone cellular components
Bone is a mineralized tissue composed of bone matrix and bone cells. Its homeostasis is mainly due to the tightly integrated contrasting activity of two major bone cell types: bone forming osteoblasts and bone resorbing osteoclasts. These cells are functionally connected and regulated by mediators such as hormones, vitamins and cytokines that strongly affect the skeletal biology throughout life [29].
Osteoblasts arise from mesenchymal stem cells and determine the formation and structural organization of bone extracellular matrix and its mineralization [30]. Mature osteoblasts synthesize several molecules involved either in bone formation or in regulating osteoclast activity such as type I collagen, osteocalcin, osteopontin, proteoglycans, receptor activator for nuclear factor κB ligand (RANKL) and osteoprotegerin (OPG) [31]. Notably, osteoblasts may also evolve to osteocytes when embedded in bone matrix, playing an important role in the control of architectural bone structure [32,33]. Osteoclasts are members of the monocyte/macrophage lineage originating from multiple cellular fusions of their precursors [34] that proliferate and differentiate towards mature osteoclasts by means of macrophage colony-stimulating factor (M-CSF) and RANKL [35]. M-CSF mainly induces precursor cell proliferation whereas RANKL plays a pivotal role in their differentiation, full functional activation and multiple cellular fusions of osteoclasts. Mature osteoclasts are able to resorb bone both by acid environment induction and secretion of lytic enzymes [36-38] such as cathepsin K and tartrate-resistant acid phosphatase (TRAP).
The functional balance and cross-talk between osteoblasts and osteoclasts are crucial in determining bone mass (Fig. 1), which depends on the well tuned bone remodelling characterized by osteoclast bone resorption and osteoblast bone rebuilding phases [31]. An imbalance of the osteoblast/osteoclast interaction due to pathological conditions such as infection, hormonal, immunological and metabolic disorders, impairs both bone mass and structure impairment resulting in increased bone fragility and fracture risk.
The role of HIV infection
As avian, feline and murine retroviruses are known to infect osteoblasts and osteocytes [39-41], early studies focused on the hypothesis that human osteoblasts may be a permissive target for HIV infection decreasing BMD through a direct viral mechanism. Some reports showed that HIV-1 transmission can occur during bone transplantation [42] and HIV-positive PCR assay has been observed in bone graft [43]. H9 cell line or peripheral blood mononuclear cells (PBMC) cocultivated with bone fragments from HIV-1-positive individuals displayed both a positive HIV RT activity and p24 detection in cell supernatants [44]. However, it was not clear whether blood or bone marrow HIV-positive cells contamination could be excluded in these studies. Mellert et al. [45] found that osteoblast-like cell lines were infected when challenged by HIV. Together, these data suggested that bone might be considered an HIV reservoir where the limited blood flow and the particular anatomical structure may also induce a poor antiretroviral concentration to tackle the HIV infection [46]. Moreover, the infection of osteoblasts may be closely related to the incomplete refilling of bone lacunae during bone remodelling with subsequent bone loss. In spite of these observations, further studies performed on human primary osteoblasts did not confirm the results obtained in osteoblast-like cell lines. The primary osteoblasts taken from HIV-positive individuals did not show viral DNA and RNA in PCR assays [47]. In addition, another study disclosed the failure of HIV productive infection when cultures of primary osteoblasts were challenged with classical HIV laboratory strains [48]. The lack of susceptibility may be partially explained by shortage of CD4 receptor and coreceptor proteins on osteoblast cell membrane [47,48]. In addition, as observed on CD34+ hematopoietic progenitor cell membrane [49], conceivably the CD4/CXCR4 complexes might be not so sterically closed to constitute the trimeric complex with gp120 essential for HIV entry.
In addition to the direct effect of HIV replication, the apoptosis process plays a pivotal role in HIV pathogenesis. The progressive loss of CD4+ T lymphocytes is also related to apoptosis activated by the interaction between HIV gp120 and the CD4 receptor [50,51]. In addition, HIV-related apoptosis is a major mechanism involved in anaemia, thrombocytopenia and induction of neuronal cell death [52-54]. A recent paper showed an increased rate of apoptosis in primary osteoblasts treated by gp120 or challenged with heat-inactivated HIV laboratory strains [48]. Apoptosis activation occurs by a paracrin/autocrin mechanism due to TNF-α increase [48]. This finding may suggest that part of the bone loss detected in HIV-infected patients may be related both to apoptosis and the decreased biological activity of osteoblasts. The inhibitory effect of HIV gp120 on osteoblast function was confirmed by Cotter et al. [55] who found that gp120 (Fig. 2 and Table 2) reduces calcium deposition, alkaline phosphatase activity and bone specific Runt-related transcription factor 2 (RUNX-2) transcription factor expression after 24 h of treatment in primary osteoblast cultures. In agreement with these data, histomorphometric and serological analysis showed impairment in primary osteoblast functional activity and a consistent decrease of serum osteocalcin in HIV-infected patients [5,56,57].
Osteoblasts are derived from bone marrow mesenchymal stem cells. Hence, some studies sought to establish whether mesenchymal stem cells and their differentiation towards osteoblasts are impaired by HIV infection (Fig. 2). Wang et al. [58] showed that bone marrow mesenchymal stem cells could be infected to a low extent by X4 tropic HIV strains leading to persistent harbouring of the virus inside these cells with subsequent inhibition of proliferation and survival. Several differentiation pathways from mesenchymal cells are also impaired by Tat through the upregulation of TNF-α and IL-1ß expression.
More recently, the interaction between specific HIV proteins and mesenchymal cells differentiating towards osteoblasts was analysed. In particular, p55gag and gp120 viral proteins elicited a derangement of specific transcription factors involved in the differentiation and activity of osteoblasts. HIV gp120 (Fig. 2) is also able to trigger the activation of peroxisome proliferator-activated receptor gamma (PPARγ) determining an MSC differentiation switch from osteoblasts to adipocytes [55,59].
Several reports have also analysed the influence of HIV on osteoclasts (Table 2). RANKL and M-CSF are key factors modulating the proliferation and differentiation of osteoclast lineage cells. A significant increase in plasma RANKL levels with an impairment of RANKL/OPG ratio was described in HIV-positive patients [60]. The RANKL increase correlated with high plasma viral RNA load indicating a direct relation between HIV infection status and RANKL synthesis [60-62]. Moreover, gp120 upregulated RANKL secretion (Fig. 3) in primary T cells [63] whereas Vpr synergized the glucocorticoid-mediated activation of RANKL in several cell systems such as primary T cells and Jurkat lymphoblastoid cell line [64]. In turn, RANKL upregulates HIV replication in acutely and chronically infected monocyte and T-lymphocyte lineages suggesting a feedback loop between HIV replication and RANKL production [65].
M-CSF is a haematopoietic growth factor controlling the survival, proliferation and differentiation of the monocyte-macrophage lineage and it is closely involved in the early phases of osteoclast differentiation. The pivotal involvement of M-CSF and its receptor in osteoclast differentiation was also confirmed by osteopetrosis and bone alterations in mice mutated in the CSF-1 or c-fms gene [66,67]. HIV infection of macrophages induces a significant increase in M-CSF production and secretion [68], which in turn promotes further HIV infection of macrophages through the increase in CD4/CCR5 receptors and virus gene expression [69-72]. M-CSF elicits osteoclast differentiation also enhancing the RANKL effect (Fig. 3). In addition, Yamada et al. [73] showed that bone marrow macrophages (BMMs) cultured without M-CSF produce a large amount of OPG compared with cells cultured with M-CSF. This finding suggests that M-CSF downregulates OPG production in BMMs. As OPG, a TNF receptor family secreting glycoprotein, inhibits osteoclast differentiation by acting as a decoy RANKL receptor, the increasing level of M-CSF during HIV infection impairs the balance between RANKL/RANK and OPG, increasing osteoclasts (Table 2).
The role of HAART
In 1995, the introduction of HAART in the treatment of HIV infection led to a dramatic and sustained decrease in HIV-related morbidity and mortality [74]. HAART typically combines nucleoside analogue reverse transcriptase inhibitors (NRTIs) with either HIV protease inhibitors or nonnucleoside reverse transcriptase inhibitors (NNRTIs). Despite controversial results regarding antiretroviral molecules and bone loss, several groups investigated the possible bone damage mechanisms of specific antiretroviral classes.
The role of N(n)RTIs
The nucleoside analogues (NRTIs) are antiretroviral molecules whose chemical structure is a modified nucleoside. These compounds suppress the replication of retroviruses by interfering with the reverse transcriptase enzyme activity causing premature termination of the proviral HIV DNA chain. Abacavir, didanosine, emtricitabine, lamivudine, stavudine, zalcitabine and zidovudine are currently used in HAART.
Despite the major positive impact of these molecules in HIV therapy, clinical observations disclosed severe side effects such as mitochondrial toxicity, hyperlactataemia and lactic acidosis. To varying degrees, NRTIs inhibit the DNA polymerase-γ [75], the enzyme involved in the replication of mitochondrial DNA, leading to mitochondrial damage and dysfunction [76]. In-vitro studies disclosed some differences in the induction of specific NRTI-related mitochondrial DNA depletion. The so-called 'd-drugs' ddC (zalcitabine), ddI (didanosine), and d4T (stavudine) are relatively stronger inhibitors of polymerase-γ than other nucleoside analogues, called 'non-D drugs' [77,78]. In the presence of mitochondrial dysfunction or depletion, the metabolism of pyruvate is shifted toward the production of lactate with a decrease in energy production. Hyperlactataemia does not inevitably lead to lactic acidosis even though this condition is more prevalent in women, obese individuals, individuals with hepatitis C virus (HCV) coinfection and patients receiving stavudine plus didanosine [79,80]. Several studies based on NRTIs-treated patients demonstrated that hyperlactataemia is a relatively common event occurring in 15-20% of individuals a year, whereas lactic acidosis is encountered in less than 0.4% of patients [79,80].
Carr et al. [13] analysed 221 patients by univariate logistic regression and found an association between NRTIs, elevated lactate levels and reduced BMD (Table 3). These data suggest that lactic acidaemia induced by NRTIs may cause osteopenia by a mechanism related to calcium hydroxyapatite loss as the bone tries to buffer chronic acidosis. This damage mainly affects the trabecular bone, which represents the majority of vertebral bone and is a more labile store of calcium than cortical bone. In addition the systemic effects of NRTIs, a paper by Pan et al. (Table 3) showed a specific interaction between zidovudine (ZDV) and bone. ZDV enhances the RANKL-mediated osteoclastogenesis inducing osteopenia in a murine model [81].
Although it is commonly classified with NRTIs, tenofovir disoproxil fumarate (TDF) is a nucleotide analogue with anti-HIV activity. In-vitro studies demonstrated that the potential of TDF to cause mitochondrial toxicity is very low compared with other NRTIs [82,83]. Preclinical animal studies have shown that renal excretion is the primary route of TDF elimination by a combination of tubular secretion and glomerular filtration and some evidence of mild nephrotoxicity was noted in different animal species [84]. This renal toxicity is dose-dependent [85], related to tubular dysfunction due to proximal tubular epithelial cell damage, and correlates with an impaired glomerular filtration rate. The tubular dysfunction may elicit the appearance of hypophosphataemia, whereas the reduced glomerular filtration is associated with a parallel decrease in the function of the alpha-1-hydroxylase, an enzyme involved in vitamin D metabolism [86]. Some papers and case reports have described a nephrotoxicity with hypophosphataemia (Table 3) in HIV-infected patients receiving TDF [87-90] and, in rare cases, the presence of Fanconi syndrome [91,92]. Fanconi syndrome was mainly observed in patients treated with salvage therapy containing ritonavir [93-95] or lopinavir/ritonavir [96]. The impaired phosphorus balance and vitamin D metabolism related to renal toxicity may determine an osteomalacic pattern in HIV patients: some studies found an association between use of TDF and bone damage [97,98] and a higher incidence of foot fracture was also found in TDF-treated patients compared with TDF-untreated individuals [99,100].
The association between TDF and nephrotoxicity was not confirmed by other studies. A cohort study published in 2006 [101] showed a low-grade hypophosphataemia in TDF-treated patients with normal baseline renal function, but it was not statistically significant with respect to TDF-untreated patients. The GS 902 [102] and GS 907 studies [103], performed on a large number of patients with no history of renal disease, found the same incidences of elevated serum creatinine and hypophosphataemia in the TDF arm and placebo arm after 24 weeks of treatment. The larger GS 903 study, a randomized, double-blind, parallel, placebo-controlled trial over 144 weeks, compared a treatment regimen of TDF, lamivudine and efavirenz with a treatment regimen of stavudine, lamivudine and EFV in antiretroviral-naive patients [104,105], confirming the GS 902 and GS 907 results.
These controversial results may be related to cohort selection, as the patients in the GS studies did not show low GFR at baseline. It is conceivable that the continuous therapeutic regimen, specific HAART pharmacological association and basal functional renal conditions of patients affected the interaction between TDF and bone.
The role of protease inhibitors
Protease inhibitors impair HIV replication by preventing the viral protease enzymatic action, a pivotal step in the final stages of the viral replication cycle. The viral progeny obtained in the presence of protease inhibitor cannot infect the target cells. Amprenavir, atazanavir, darunavir, fosamprenavir, indinavir, lopinavir, nelfinavir, ritonavir, saquinavir and tipranavir are the protease inhibitors used for antiretroviral therapy.
The protease inhibitors/bone interaction has been studied in in-vitro bone cell cultures (Table 3). The effects of protease inhibitors on osteoclasts were studied measuring osteoclast activity in rat neonatal calvaria [106]. Nelfinavir, indinavir, saquinavir and ritonavir treatments showed proosteoclast activity whereas lopinavir and amprenavir did not. Further studies demonstrated that saquinavir and ritonavir improved osteoclast activity through the abrogation of a physiological block to RANKL signalling represented by interferon gamma (IFN-γ)-mediated proteosomal degradation of TNF receptor associated factor 6 (TRAF-6) [63]. RANKL recruits signal adapter TRAF-6 to the cytoplasmic tail of RANK resulting in the activation of nuclear factor kappa-light-chain-enhancer of activated B cells (NF-κB) and mitogen-activated protein kinase (MAPKinase) pathways [107] involved in the survival and differentiation of osteoclasts. A subsequent paper [108] showed that ritonavir had opposite effects blocking osteoclastogenesis by impairing RANKL-induced signals. This finding suggested a complex scenario in the interactions between protease inhibitors and the osteoclast lineage. Protease inhibitors were also assayed on the human mesenchymal stem cells differentiating to osteoblast lineage. These experiments indicated that nelfinavir and lopinavir inhibited bone formation and calcium deposition thereby decreasing osteoblast activity [63]. A recent paper by Malizia et al. [109] assayed four protease inhibitors (nelfinavir, saquinavir, indinavir, ritonavir) on primary osteoblasts and found a significantly decreased osteoblast activity (decrease of alkaline phosphatase, calcium deposition and RUNX-2 mRNA expression) when indinavir and ritonavir were used. Altogether these in-vitro studies suggest that some protease inhibitors may determine bone loss by increasing osteoclast resorption and inhibiting the osteoblast rebuilding function.
It is noteworthy that some in-vitro studies investigated the possible association between use of protease inhibitors and decreased vitamin D serum levels. Vitamin D is essential for the maintenance of a normal bone structure increasing the phosphate bone bioavailability [110]. The biological effects on bone remodelling are exerted by 1,25-dihydroxyvitamin D3 (calcitriol), a potent calcitropic hormone [111]. Vitamin D activation to calcitriol involves 25-hydroxylation in the liver followed by 1α -hydroxylation of 25-hydroxyvitamin D3 in the renal proximal tubular cells, whereas vitamin D catabolism is mainly determined by 24-hydroxylase. The vitamin D deficit can progressively determine osteomalacia through the reduction of phosphates available to bone [112].
The 1α-hydroxylase and 25-hydroxylase enzymes, involved in vitamin D activation, are cytochrome P450 monoxygenases [111] and protease inhibitors are potent in-vivo inhibitors of human hepatic cytochrome P450s namely CYP3A4 [113-115]. Ritonavir, but also indinavir and nelfinavir, negatively affect the 1α-hydroxylase enzyme and, to a lesser extent, 25-hydroxylase enzyme activity reducing 1,25-dihydroxyvitamin D3 production (Table 3) whereas no inhibition of 24-hydroxylase is observed [115,116].
Some clinical studies investigated vitamin D deficiency in HIV-infected individuals. Hypovitaminosis D was observed before the advent of HAART and a severe depletion of vitamin D was associated with advanced infection and immune system hyperactivation [117]. Recent studies performed on a cohort of naive and HAART-treated patients demonstrated a high prevalence of hypovitaminosis D suggesting a risk of osteomalacia [118,119]. These results, coupled with the TDF effects on vitamin D regulation indicate that HAART-mediated osteomalacia may be an additional mechanism of bone depletion. Osteomalacia could be underestimated as a pathogenetic event of bone impairment in HIV infection. This problem is not fully appreciated in the literature, because the few studies that have tried to explore bone impairment have usually used the DXA scan. This procedure is a good approach to determine bone mineral content but cannot discriminate between osteoporosis (low BMD and bone architecture deterioration) and osteomalacia (low BMD with normal bone architecture).
Management of osteopenia/osteoporosis in HIV-infected patients
Diagnosis
In daily clinical practice, doctors must address many problems affecting their HIV-infected patients, such as the prevention of cardiovascular disease, diabetes, dyslipidaemia and lipodystrophy. Although specific complete guidelines are not yet available, several diagnostic approaches have been recommended to monitor bone conditions during HIV disease and the HAART regimen (Table 4).
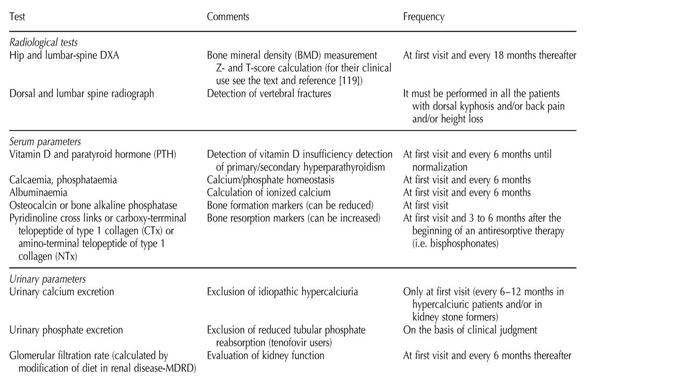
Hip and lumbar-spine DXA analysis is a valuable approach to determine BMD variations and will discriminate between cortical and trabecular bone, two different compartments that may respond differently to antiretroviral drugs. Hence, DXA should be performed in all patients, at least at baseline visit, as many prospective studies have demonstrated that it will predict fracture risk [9]. A widely cited meta-analysis indicated that the risk of hip fracture increased 2.6-fold for each standard deviation decrease in BMD at the femoral neck [120]. Unfortunately, DXA machines are not widely available and many doctors cannot prescribe the analysis easily, but a major effort should be made to obtain at least one BMD measurement in all HIV-infected patients.
The recent guidelines devised by the International Society for Clinical Densitometry (ISCD) recommend using the T-score with the diagnostic cut-off value specified by WHO only for women in menopause. Although definitive data are lacking, it is generally accepted that the same method can be applied to men over the age of fifty if they have at least one major risk factor for osteoporosis. For individuals aged less than 50 years, diagnosis is recommended using the Z-score that compares the patient's BMD with that of a healthy age-matched and sex-matched population. However, the Z-score has no clear cut-off value for osteopenia and osteoporosis and the following scores are recommended: patients with values lower than -1 are classified as having low bone mass, whereas a severe bone mass reduction is identified by Z-score values lower than -2 [121]. The Z-score may allow further in-depth analysis of BMD and a useful application of this parameter in the evaluation of bone loss in HIV-infected individuals. Osteomalacia can only be diagnosed by bone histomorphometry that will disclose large amounts of unmineralized bone matrix. As bone biopsies are invasive, the diagnosis of osteomalacia is indirect and is generally established by coupling DXA analysis with some blood analytes (i.e. vitamin D, calcium, phosphate, PTH). Hence, DXA alone may underestimate the osteomalacia rate in this population as an unknown number of patients may have been misdiagnosed with osteoporosis. Nonetheless, a correct diagnosis must be established in order to institute appropriate therapy. Osteoporosis is commonly treated with antiresorptive or anabolic agents [9] whereas osteomalacia requires high doses of vitamin D [112].
Dorsal and lumbar spine radiograph are useful to assess vertebral fractures. Radiological investigation must be entertained in patients with back pain (particularly occurring in the upright position), patients with marked height reduction and in patients with severe kyphosis; at follow-up spine radiograph must rely upon the physician's decision particularly in patients with a diagnosis of vertebral fractures. Bone biology parameters can be monitored by laboratory tests: calcaemia, phosphataemia and albuminaemia (for ionized calcium calculation), urinary calcium and phosphate excretion. In addition, bone formation markers (i.e. osteocalcin or bone-associated alkaline phosphatase) and bone resorption markers (pyridinoline cross links or CTx, or NTx) can yield useful information together with vitamin D and PTH assays. Important information may be obtained by GFR determination (age and TDF, together with a low GFR, inducing a decrease in the function of alpha-1-hydroxylase in the kidney and in vitamin D availability) and the evaluation of kidney tubular function (some proximal tubular alterations may occur with normal GFR, and may signify an initial impairment of tubular function potentially unsafe for bone). Plasma lactic acidaemia monitoring at baseline and periodically during follow-up may provide valuable information on the side effects of NRTIs on bone. Mora et al. [62] suggested that plasma RANKL and OPG determination may be employed in some cases of therapy evaluation, as impairment of the RANKL/OPG system is well described in patients receiving protease inhibitor-based HAART. Even though short-term data indicate that replacing stavudine and protease inhibitor with tenofovir and efavirenz restores the RANKL/OPG equilibrium and may thus lead to a reduction in the bone resorption rate. The determination of these cytokines is not yet part of the routine evaluation of HIV-infected patients.
Therapy
The management of osteopenia/osteoporosis in the course of HIV infection may be based on a change in risk factors, calcium and vitamin D diet supplementation and biphosphonate drugs (Table 5).
Patients can be advised to stop smoking and take physical exercise to control body weight. In addition, patients should have correct diet indications to ensure an adequate uptake of calcium and vitamin D. However, cholecalciferol should be administered to all HIV-infected patients presenting vitamin D deficiency. The daily upper tolerable limit for vitamin D is fixed at 2000 international unit (IU), but this is far below the toxic threshold, that has never been reached even with the administration of 10000 IU per day [122]. A supplementation of 800 IU cholecalciferol daily can be suggested to all outpatients. The only contraindication to cholecalciferol administration is hypercalcaemia and therefore when serum ionized calcium is normal, vitamin D can be used.
A low calcium diet has been demonstrated to reduce BMD and maybe to increase the hip fracture risk [123,124]. The daily calcium recommended allowance for adults is between 800 and 1000 mg per day [125], but it is very difficult to reach this threshold in cholesterol lowering diets. Therefore, it is very important for correct diet counselling to be given to all HIV-infected patients.
When vitamin D deficiency and low calcium intake have been corrected, drugs can be used. Many treatments are available to combat osteoporosis. The antifracture efficacy, at least for vertebral fractures, is well demonstrated for bisphosphonates (alendronate, risedronate, ibandronate, and zoledronate), hormone replacement therapy, raloxifene, strontium ranelate, teriparatide and recombinant human parathyroid hormone (PTH) [9].
Four studies have been published on the pharmacological treatment of HIV-induced osteoporosis with alendronate. Due to the low number of individuals analysed, the results obtained have a limited statistical significance, even though they showed that alendronate increased BMD with respect to placebo [126-129]. More recently, similar results were obtained in two trials comparing once yearly zoledronate therapy with placebo [130,131]. No data are available on fracture incidence reduction yet. Further studies are necessary to investigate the effects of bisphosphonates both on BMD and fracture risk reduction in HIV-related bone disease. Nevertheless, especially in fractured patients, the risk of new fractures is very high and therefore this therapeutic approach is mandatory.
The advantages and disadvantages of bisphosphonate treatment must be clearly evaluated for each patient. Notwithstanding the scant data available, alendronate or zoledronate must be considered to enhance bone mineral density and possibly decrease fracture incidence. The adverse effects associated with bisphosphonates include gastrointestinal intolerance (with oral administration), short-lived acute phase reaction (with intravenous administration) and jaw osteonecrosis [132], but the relatively low risk of this last adverse effect does not contraindicate the use of bisphosphonates at present.
A possible future direction in the treatment of osteoporosis may be the use of the anti-RANKL monoclonal antibody (denosumab). As described earlier, RANKL induces osteoclast activation and its upregulation was noted both in HIV-positive [60-62] and HIV-negative patients with osteopenia/osteoporosis [133]. A clinical study on 412 HIV-negative postmenopausal women given denosumab for 1 year demonstrated an increase in BMD and a decrease of bone turnover [134]. This finding suggested its possible use in osteoporosis treatment even in HIV-positive individuals. Other compounds such as OPG (RANK/RANKL interaction inhibitor), raloxifene (selective estrogen receptor modulator), teriparatide (PTH analogue), PTH and strontium ranelate (dual action bone agent) are under study but, like denosumab (RANK/RANKL interaction inhibitor), further evaluation is needed before these drugs can be administered in HIV-infected patients (Table 6).
Conclusion
Bone derangement is a major clinical complication in the course of HIV infection [135]. The advent of HAART has led to a longer life expectancy and therefore HIV/HAART-related bone disease is destined to increase, enhancing the physiological age-related bone loss. Some epidemiological surveys in HIV-uninfected individuals indicate that the percentage of osteoporosis after 45 years of age ranges between 10 and 20%, and the lifetime fracture risk for a 50-year-old White woman is greater than 50% [136,137]. These data suggest that the number of HIV patients with bone disease and silent fractures can be expected to increase dramatically in the next few years because these patients also have two other potentially worsening factors: HIV itself and antiretroviral therapy. Hence, antiretroviral therapy must be accompanied by the clinical management of HIV/HAART-related bone disease to reduce the risk of osteoporosis and fractures in these patients.
|
|
|
|
|
|
|